By Lindsay Wickman, Postdoctoral Scholar, Oregon State University Department of Fisheries, Wildlife, and Conservation Sciences, Geospatial Ecology of Marine Megafauna Lab
Earlier this month, most of the GEMM Lab and I attended the 25th Biennial Conference on the Biology of Marine Mammals in Perth, Western Australia. This year’s theme, “Fishing for Change,” acknowledged that incidental entanglement in fishing gear is currently the most pervasive threat to marine mammals (e.g., Avila et al., 2018). While many presentations on the prevalence and impacts of entanglement on marine mammals were sobering, it was also inspiring to be surrounded by so many dedicated people working to address this urgent issue. For me, one of the most memorable anecdotes was an incredible whale disentanglement story shared by Paul Cottrell, a Marine Mammal Coordinator at the Department of Fisheries and Oceans Canada (DFO) in British Columbia.
An Incredible Story of Whale Disentanglement
During August 2024, DFO and a local NGO (Straitwatch) responded to a report of two humpback whales entangled in the same fishing gear near Quadra Island, B.C., Canada. Their photo-identification histories revealed that one whale had migrated from Hawaii, while the other had come from Mexico. Now tied together, the two whales’ fates became intertwined, forcing them to coordinate their movements. This situation obviously raised concerns about their welfare and survival, but I also had to silently wonder, “Did the two whales ever argue about where to migrate next? Would they choose Hawaii or Mexico?”
Thanks to the rescuers’ efforts, both whales were freed and able to make their own choice about where to spend the breeding season. As Paul explained, successfully disentangling one whale is challenging and dangerous, so freeing two was an impressive feat. After the rescue, a video showed the whales continuing to swim together synchronously, as if they did not realize they were no longer connected!
Most Entangled Whales are Out of Sight
The story above exemplifies a “confirmed” entanglement—these whales were seen dragging fishing gear and the event was reported by concerned citizens. However, most entanglement events are never witnessed, for several reasons.
When a whale becomes entangled in fishing gear, it rarely remains anchored in place. Instead, the whale often breaks part of the gear, dragging it behind as it swims. The likelihood of observing the entangled whale subsequently depends on both the chance of it being seen and the observer’s awareness and willingness to report the event (Robbins and Mattila, 2004).
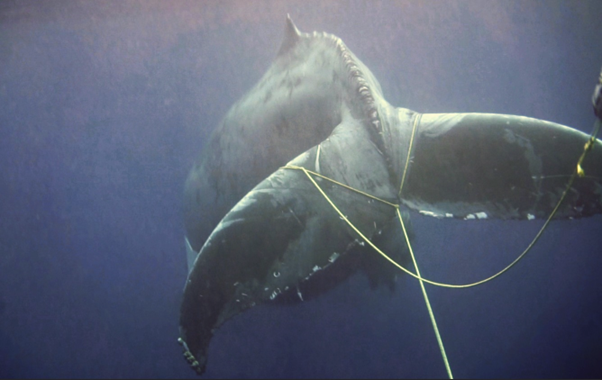
Once entangled, many become a “dead whale swimming,” eventually succumbing to starvation and/or infections (Dolman and Moore, 2017). Many entanglements involve the mouth, severely impacting the whale’s ability to feed (Moore and van der Hoop, 2012). The additional drag imposed by entanglement is comparable to the energetic costs of migration or reproduction, causing a significant depletion in their energy reserves (van der Hoop et al. 2015). Serious injuries include amputations, hemorrhage, and infections (Cassoff et al., 2011).
Although some carcasses of entangled whales wash ashore, most are lost at sea and never recovered. For example, even with relatively intensive monitoring for North Atlantic right whale (NARW) carcasses, Pace et al. (2021) estimated that recovered carcasses represented just 36% of the total deaths. These recovered carcasses may also underestimate the toll of entanglement; entanglement accounted for 51% of mortality in the carcasses vs. 87% of serious injuries observed in living NARWs (Pace et al., 2021).
For whales that manage to dislodge the gear and survive, scars can provide clues to their past entanglement history. Injuries from the fishing lines can leave indentations where they cut through skin and blubber, and healed wounds often result in white pigmented scars that wrap around the body (especially the flukes and peduncle; e.g., Robbins and Matilla 2004). The widespread prevalence of these scars suggests that in many cases, whales can actually dislodge the gear on their own. For example, a study of entanglement scars on humpback whales in the Gulf of Maine revealed that 10% of adults and 30% of juveniles acquired new entanglement scars between 2009-2010. Without scarring analyses though, most of these entanglements would have been missed; just 7% of these individuals with entanglement-related scars were seen while entangled (Robbins 2012).
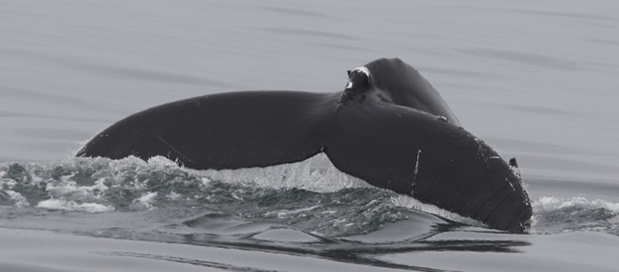
Unfortunately, scars are not the only long-term consequence of non-lethal entanglement events. Previously entangled NARWs have lower survival rates than unaffected individuals (Robbins et al. 2015, Reed et al. 2024), and long-term stress responses can impact their future health and reproductive success (Pettis et al. 2004). It is tempting to assume that only severe entanglements affect future reproduction and survival, but a lack of extensive external injuries doesn’t necessarily mean that the impact of the entanglement event is more minor (Robbins and Matilla, 2004). For example, Reed et al. (2024) found that NARWs with entanglement injuries classified as minor were less likely to transition from a “non-breeder” to “breeder” status than those with severe injuries.
Tracking Unseen Entanglements: Project SLATE
Since reported entanglements and recovered carcasses reveal just a fraction of actual entanglements, researchers are continuing to innovate ways of documenting these “unseen” entanglement events.
As discussed in a previous blog post, photos of entanglement scars on the flukes and peduncles of humpback whales are being utilized in Project SLATE to detect trends in entanglement off the coast of Oregon, USA. Analyzing images of whales for signs of past entanglements is a meticulous process that may not seem as thrilling as responding to an actual disentanglement event. However, in areas with lower population densities, such as the Oregon coast, reported entanglements are undoubtedly an underestimate of the true number of events. Thus, tracking scarring rates can provide more comprehensive data on entanglement prevalence in Oregon than confirmed reports alone.
What to do if you see an entangled whale
If you happen to observe an entangled whale, please do not attempt to disentangle it yourself. Whale disentanglement is dangerous and complex, so best left to the experts! When well-meaning citizens attempt a disentanglement on their own, it can also result in an “incomplete disentanglement,” where some, but not all, gear is removed from the whale. Incomplete disentanglements just make it harder for responders to subsequently find and successfully rescue the whale.
Instead, report the entanglement by promptly calling:
- Entanglement Reporting Hotline: 1-877-SOS-WHAL or 1-877-767-9425
- or U.S. Coast Guard: VHF Ch. 16
Videos or photos showing the entangling gear is very helpful to trained responders, but remember to stay at least 100 yards from the whale, and beware of snagging your vessel in the lines. Visit NOAA Fisheries for more information.
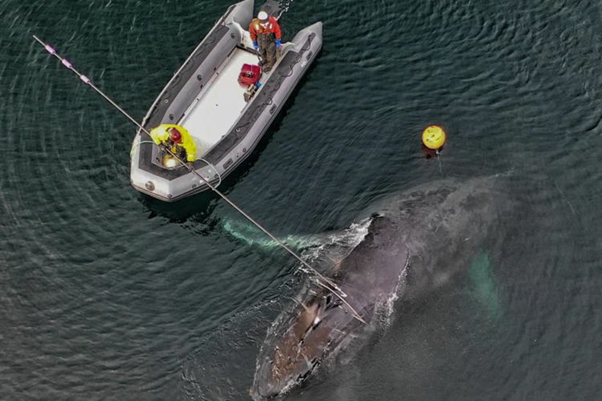
References:
Avila, I.C., Kaschner, K., Dormann, C.F.. (2018). Current global risks to marine mammals: Taking stock of the threats. Biol. Conserv. 221, 44–58.
Cassoff, R.M., Moore, K.M., McLellan, W.A., Barco, S.G., Rotstein, D.S., Moore, M.J. (2011). Lethal entanglement in baleen whales. Dis. Aquat. Organ. 96, 175–185.
Dolman, Sarah J., and Michael J. Moore. (2024). Chapter 4: Welfare implications of cetacean bycatch and entanglements. In A. Butterworth (Ed.) Marine Mammal Welfare: Human Induced Change in the Marine Environment and Its Impacts on Marine Mammal Welfare (pp. 41-65).
Moore, M. J., and van der Hoop, J. M. (2012). The painful side of trap and fixed net fisheries: chronic entanglement of large whales. Journal of Marine Sciences, 2012.
Pace III, R. M., Williams, R., Kraus, S. D., Knowlton, A. R., & Pettis, H. M. (2021). Cryptic mortality of North Atlantic right whales. Conservation Science and Practice, 3(2), e346
Reed, J., New, L., Corkeron, P., Harcourt, R. (2024). Disentangling the influence of entanglement on recruitment in North Atlantic right whales. Proc. R. Soc. B Biol. Sci. 291.
Robbins, J., Mattila, D. (2004). Estimating humpback whale (Megaptera novaeangliae) entanglement rates on the basis of scar evidence. Rep. to Northeast Fish. Sci. Center, Natl. Mar. Fish. Serv. 43EANF030121 22p.
Robbins, J. (2012). Scar-Based Inference Into Gulf of Maine Humpback Whale Entanglement : 2010. Report to the Northeast Fisheries Science Center National Marine Fisheries Service, EA133F09CN0253 Item 0003AB, Task 3.
van der Hoop JM, Corkeron P, Kenney J, Landry S, Morin D, Smith J, Moore MJ. (2015). Drag from fishing gear entangling North Atlantic right whales. Mar Mamm Sci 32(2):619–642.