Clara Bird, PhD Student, OSU Department of Fisheries and Wildlife, Geospatial Ecology of Marine Megafauna Lab
Greetings from the NOAA research vessel Shimada! As you may know from previous blogs, usually one member of the GEMM Lab goes on the Northern California Current (NCC) ecosystem survey cruises as a marine mammal observer to collect data for the project Where are whales in Oregon waters? But for this September 2020 cruise we have two observers on-board. I’m at-sea with fellow GEMM lab student Dawn Barlow to learn the ropes and procedures for how we collect data. This research cruise is exciting for a few reasons: first, this is my first cruise as a marine mammal observer! And second, this is the first NOAA Northwest Fisheries Science Center research cruise since the COVID-19 pandemic began in the United States.
Our job as observers involves surveying for marine mammals from the flying bridge, which is the upper most deck of the ship, above the Bridge where the officers command the vessel. Here, we are referred to as the “birds in the nest” by the officers (something I find fitting given my last name). We spend our time looking out at the water with our binoculars searching for any sign of a marine mammal. These signs include: a blow, a fluke, a flipper, or the splash of a dolphin. Surveys involve long stretches of time staring at the ocean seeing nothing but blue waves, punctuated by exciting moments. The level of excitement of these moments can range from finally seeing a blow in the distance to seeing a whale breach! As of the time I’m writing this blog, we’ve been at sea for six days and have four more to go, so I will describe the things we’ve seen and my experience being on a primarily oceanographic research cruise.
We started day one transiting offshore of Newport, right into some whale soup! What started as a few distant blows quickly became an ocean full of whales. Dawn and I were some-what frantic as we worked to keep track of the many humpback and blue whales that were around us (I saw my first blue whale!). We even saw a humpback whale breaching! This introduction to marine mammal observation was an exciting exercise in keeping track of blows and rapid species identification. Day two was pretty similar, as we spent the day travelling back inshore along the same path we had followed offshore on day one. It was cool to see that there were still many whales in the same area.
On day three we woke up to dense fog, and overall pretty poor conditions for marine mammal observing. One of the challenges of this work is that not only does bad weather make it hard to see, but it also makes it hard for us to be able to say that mammals were truly absent. In bad observation conditions we cannot know if we did not see anything because the animals were not there or if we just could not see them through the swell, fog or white-caps. However, by the late afternoon the fog cleared and we had a spectacular end of the day. We saw a killer whale breach (Image 1) and a humpback whale tail lobbing (smacking it’s fluke against the surface of the water) in front of a stunning sunset (Image 2).

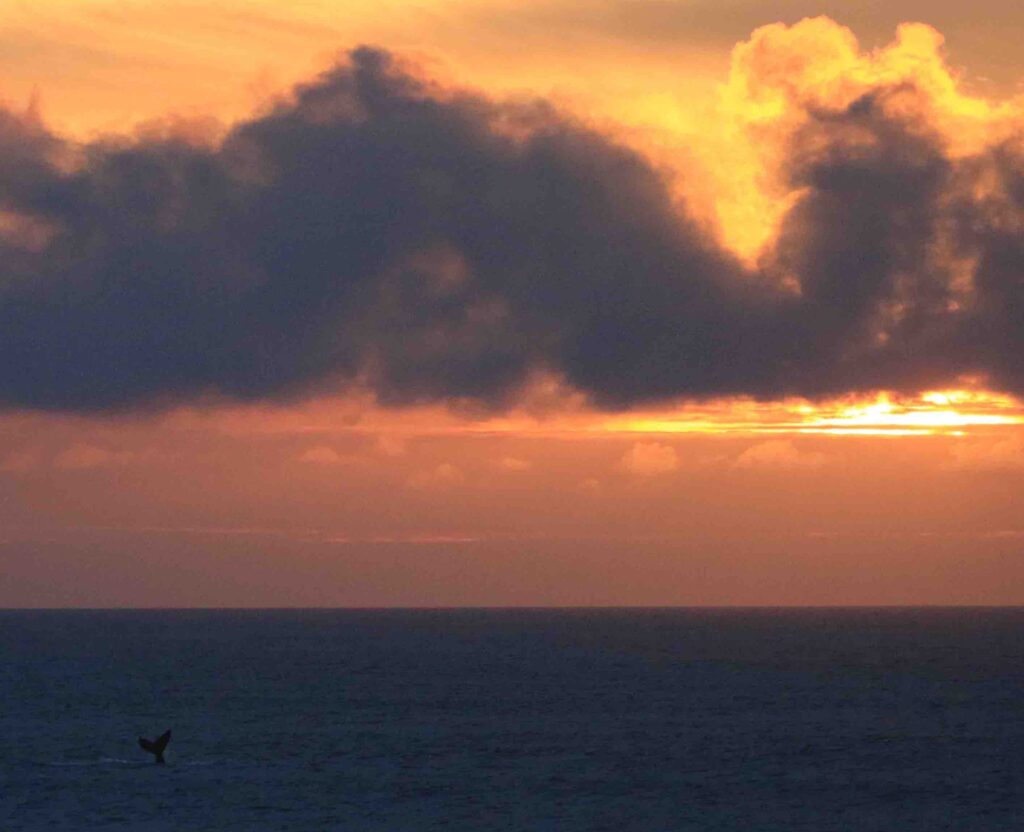
Day four was a bit of a marine mammal work reality check. While spectacular evenings like day three are memorable and exhilarating, they tend to be rare. The weather conditions on day four were pretty poor and we ended up surveying from the bridge for most of the day and not seeing much. Conditions improved on day five and we had some fun dolphin sightings where they came and rode on the wake from the bow, and observed a sperm whale blow in the distance!
The weather was not great today (day six), especially in the morning, but we did have one particularly exciting sighting right along the edge of Heceta Bank. While we were stopped at an oceanographic sampling station, we were visited by a group of ~30 pacific white sided dolphins who spent about half an hour swimming around the ship. We also saw several humpback whales, a fur seal, and a Mola mola (Ocean sunfish)! It was incredible to be surrounded by so many different species, all so close to the ship at the same time.
Overall, it has been wonderful to be out at sea after the many isolating months of COVID. And, it has been an exciting and interesting experience being surrounded by non-whale scientists who think about this ecosystem from a different perspective. This cruise is focused on biological oceanography, so I have had the great opportunity to learn from these amazing scientists about what they study and what oceanographic patterns they document. It’s a good reminder of our interconnected research. While it’s been cool to observe marine mammals and think about something totally different from my research on gray whale behavior, I have also enjoyed finding the similarities. For example, just last night I had a conversation with a graduate student researching forams (check out this link to learn more about these super cool tiny organisms!). Even though the organisms we study are polar opposites in terms of size, we actually found out that we had a good bit in common because we both use images to study our study species and have both encountered similar unexpected technical challenges in our methods.
I am thoroughly enjoying my time being one of the “birds in the nest”, contributing data to this important project, and meeting these wonderful scientists. If you are curious about how the rest of the cruise goes, make sure to check out Dawn’s blog next week!