By Rachel Kaplan, PhD student, Oregon State University College of Earth, Ocean, and Atmospheric Sciences and Department of Fisheries, Wildlife, and Conservation Sciences, Geospatial Ecology of Marine Megafauna Lab
Early June marked the onset of El Niño conditions in the Pacific Ocean , which have been strengthening through the fall and winter. For Oregonians, this climate event means unseasonably warm December days, less snow and overall precipitation (it’s sunny as I write this!), and the potential for increased wildfires and marine heatwaves next summer.
This phenomenon occurs about every two to seven years as part of the El Niño Southern Oscillation (ENSO), a cyclical rotation of atmospheric and oceanic conditions in the Pacific Ocean that is initiated by departures from and returns to “normal conditions” at the equator. Typically, the trade winds blow warm water west along the equator, and El Niño occurs when these winds weaken or reverse. As a result, the upwelling of cold water at the equator ceases, and warm water flows towards the west coast of the Americas, rather than its typical pathway towards Asia. When the trade winds resume their normal direction, usually after months or a year, the system returns to “normal” conditions – or, it can enter the cool La Niña part of the cycle, in which the trade winds are stronger than normal. “El Niño de Navidad” was named by South American fisherman in the 1600s because this event tends to peak in December – and El Niño is clearly going to be a guest for Christmas this year.
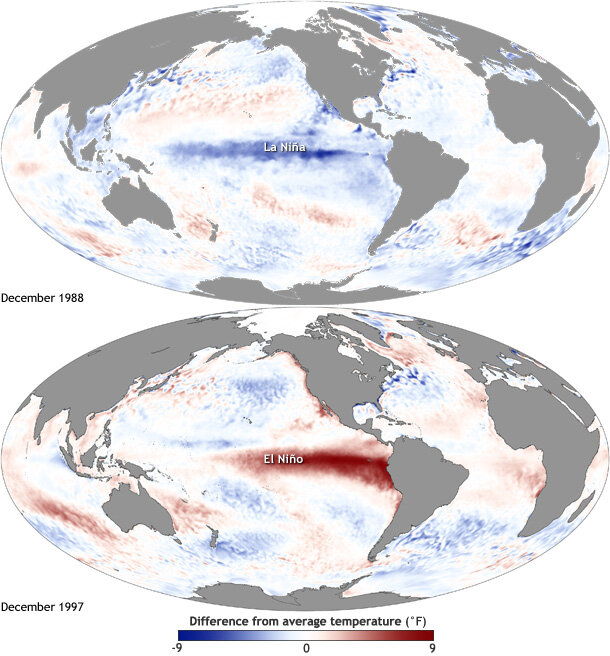
These events at the equator trigger changes in global atmospheric circulation patterns, and they can shape weather around the world. Teleconnection, the coherence between meteorological and environmental phenomena occurring far apart, is to me one of the most incredible things about the natural world. This coherence means that the biological community off the Oregon coast is strongly impacted by events initiated at the equator, with consequences that we don’t yet fully understand.
The effects of El Niño are diverse – floods in some places, droughts in others – and their onset can mean wildly different things for Oregon, Peru, Alaska, and beyond. As we tap our fingers waiting to be able to ski and snowboard in Oregon, what does our current El Niño event mean for the life in the waters off our coast?
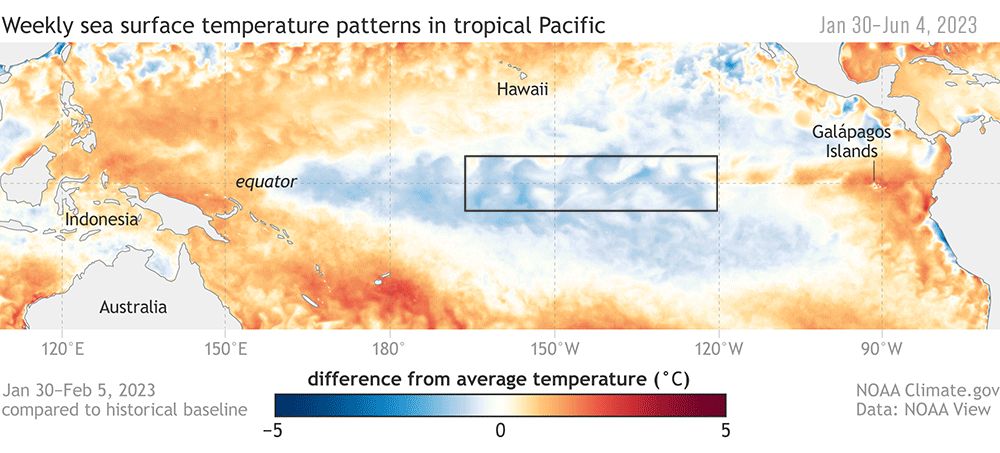
ENSO plays a big role in the variability in our local Northern California Current (NCC) system, and the outcomes of these events can differ based on the strength and how the signal propagates through the ocean and atmosphere (Checkley & Barth, 2009). Large-scale “coastal-trapped” waves flowing alongshore can bring the warm water signal of an El Niño to our ocean backyard in a matter of weeks. One of the first impacts is a deepening of the thermocline, the upper ocean’s steep gradient in temperature, which changes the cycling of important nutrients in the surface ocean. This can result in a decrease in upwelling and primary productivity that sends ramifications through the food web, including consequences for grazers and predators like zooplankton, marine mammals, and seabirds (Checkley & Barth, 2009).
In addition to these ecosystem effects that result from local changes, the ocean community can also receive new visitors from afar, and see others flee . For krill, the shrimp-like whale prey that I spend a lot of my time thinking about, community composition can change as subtropical species typically found off southern and Baja California are displaced by horizontal ocean flow, or as resident species head north (Lilly & Ohman, 2021).
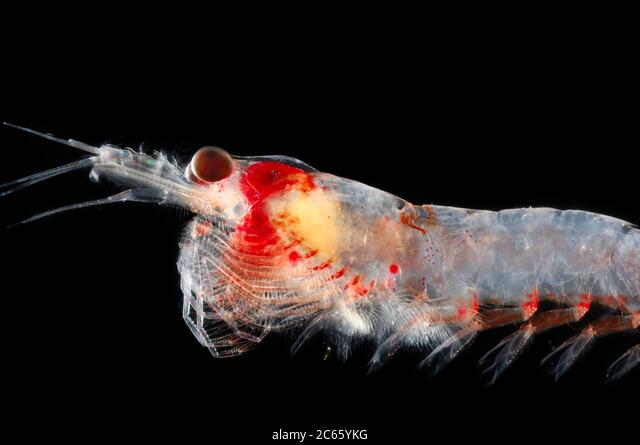
The two main krill species that occur in the NCC, Euphausia pacifica and Thysanoessa spinifera, favor the cool, coastal waters typical off the coast of Oregon. During El Niño events, E. pacifica tends to contract its distribution inshore in order to continue occupying these conditions, increasing its spatial overlap with T. spinifera (Lilly & Ohman, 2021). In addition, both tend to shift their populations north, toward cooler, upwelling waters (Lilly & Ohman, 2021).
These krill species are a favored prey of rorqual whales, and the coast of Oregon is an important foraging ground for humpback, blue, and fin whales. Predators tend to follow their prey, and shifting distributions of these krill species may cause whales to move, too. During the 2014-2015 “Blob” event in the Pacific Ocean, a marine heatwave was exacerbated by El Niño conditions. Humpback whales in central California shifted their distributions inshore in response to sparse offshore krill, increasing their overlap with fishing gear and leading to an increase in entanglement events (Santora et al., 2020). Further north, these conditions even led humpback whales to forage in the Columbia River!
As El Niño events compound with the impacts of global climate change, we can expect these distributional shifts – and perhaps surprises – to continue. By the year 2100, the west coast habitat of both T. spinifera and E. pacifica will likely be constrained due to ocean warming – and when El Niños occur, this habitat will decrease even further (Lilly & Ohman, 2021). As a result, the abundances of both species are expected to decrease during El Niño events, beyond what is seen today (Lilly & Ohman, 2021). This decline in prey availability will likely present a problem for future foraging whales, which may already be facing increased environmental challenges.
Understanding connections is inherent to the field of ecology, and although these environmental dependencies are part of what makes life so vulnerable, they can also be a source of resilience. Although humans have known about ENSO for over 400 years, the complex interplay between nature, anthropogenic systems, and climate change means that we are still learning the full implications of these events. Just as waiting for Santa Claus always keeps kids guessing, the dynamic ocean keeps surprising us, too.
References
Checkley, D. M., & Barth, J. A. (2009). Patterns and processes in the California Current System. Progress in Oceanography, 83(1–4), 49–64. https://doi.org/10.1016/j.pocean.2009.07.028
Lilly, L. E., & Ohman, M. D. (2021). Euphausiid spatial displacements and habitat shifts in the southern California Current System in response to El Niño variability. Progress in Oceanography, 193, 102544. https://doi.org/10.1016/j.pocean.2021.102544
Santora, J. A., Mantua, N. J., Schroeder, I. D., Field, J. C., Hazen, E. L., Bograd, S. J., Sydeman, W. J., Wells, B. K., Calambokidis, J., Saez, L., Lawson, D., & Forney, K. A. (2020). Habitat compression and ecosystem shifts as potential links between marine heatwave and record whale entanglements. Nat Commun, 11(1), 536. https://doi.org/10.1038/s41467-019-14215-w