By Dr. Dawn Barlow, Postdoctoral Scholar, OSU Department of Fisheries, Wildlife, and Conservation Sciences, Geospatial Ecology of Marine Megafauna Lab
The world is warming. Ocean ecosystems are experiencing significant and rapid impacts of climate change. However, the cascading effects on marine life are largely unknown. Thus, it is critical to understand how – not just if – environmental change impacts the availability and quality of key prey species in ocean food webs, and how these changes will impact marine predator health and population resilience. With these pressing knowledge gaps in mind, we are thrilled to launch a new project “Marine predator and prey response to climate change: Synthesis of Acoustics, Physiology, Prey, and Habitat in a Rapidly changing Environment (SAPPHIRE).” We will examine how changing ocean conditions affect the availability and quality of krill, and thus impact blue whale behavior, health, and reproduction. This large-scale research effort is made possible with funding from the National Science Foundation.
The SAPPHIRE project takes place in the South Taranaki Bight (STB) region of Aotearoa New Zealand, and before diving into our new research plans, let’s reflect briefly on what we know so far about this study system based on our previous research. Our collaborative research team has studied blue whales in the STB since 2013 to document the population, understand their ecology and habitat use, and inform conservation management. We conducted boat-based surveys and used hydrophones to record the underwater soundscape, and found the following:
- Blue whales in Aotearoa New Zealand are a unique population, genetically distinct from all other known populations in the Southern Hemisphere, with an estimated population size of 718 (95% CI = 279 – 1926).1
- Blue whales reside in the STB region year-round, with feeding and breeding vocalizations detected nearly every day of the year.2,3
- Wind-driven upwelling over Kahurangi shoals moves a plume of cold, nutrient-rich waters into the STB, supporting aggregations of krill, and thereby critical feeding opportunities for blue whales in spring and summer.4–6
- We developed predictive models to forecast blue whale distribution up to three weeks in advance, providing managers with a real-time tool in the form of a desktop application to produce daily forecast maps for dynamic management.7
- During marine heatwaves, blue whale feeding activity was substantially reduced in the STB. Interestingly, their breeding activity was also reduced in the following season when compared to the breeding season following a more productive, typical foraging season. This finding indicates that shifting environmental conditions, such as marine heatwaves and climate change, may have consequences to not just foraging success, but the population’s reproductive patterns.3
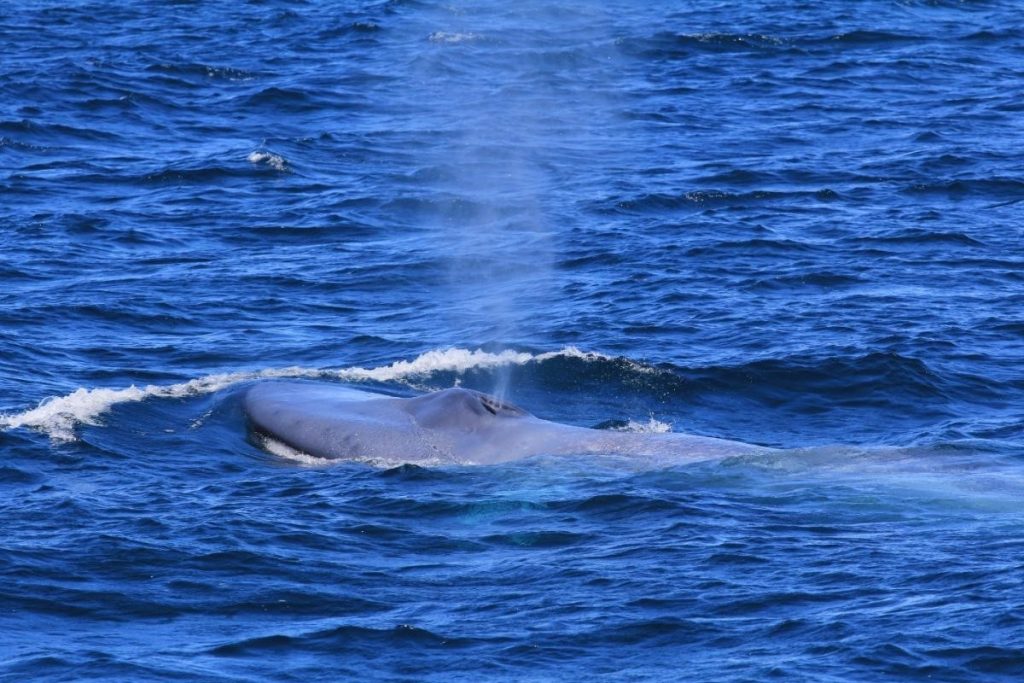
Project goals
Building on this existing knowledge, we aim to gain understanding of the health impacts of environmental change on krill and blue whales, which can in turn inform management decisions. Over the next three years (2024-2026) we will use multidisciplinary methods to collect data in the field that will enable us to tackle these important but challenging goals. Our broad objectives are to:
- Assess variation in krill quality and availability relative to rising temperatures and different ocean conditions,
- Document how blue whale body condition and hormone profiles change relative to variable environmental and prey conditions,
- Understand how environmental conditions impact blue whale foraging and reproductive behavior, and
- Integrate these components to develop novel Species Health Models to predict predator and prey whale population response to rapid environmental change.
Kicking off fieldwork
This coming January, we will set sail aboard the R/V Star Keys and head out in search of blue whales and krill in the STB! Five of our team members will spend three weeks at sea, during which time we will conduct surveys for blue whale occurrence paired with active acoustic assessment of krill availability, fly Unoccupied Aircraft Systems (UAS; “drones”) over whales to determine body condition and potential pregnancy, collect tissue biopsy samples to quantify stress and reproductive hormone levels, deploy hydrophones to record rates of foraging and reproductive calls by blue whales, and conduct on-board controlled experiments on krill to assess their response to elevated temperature.
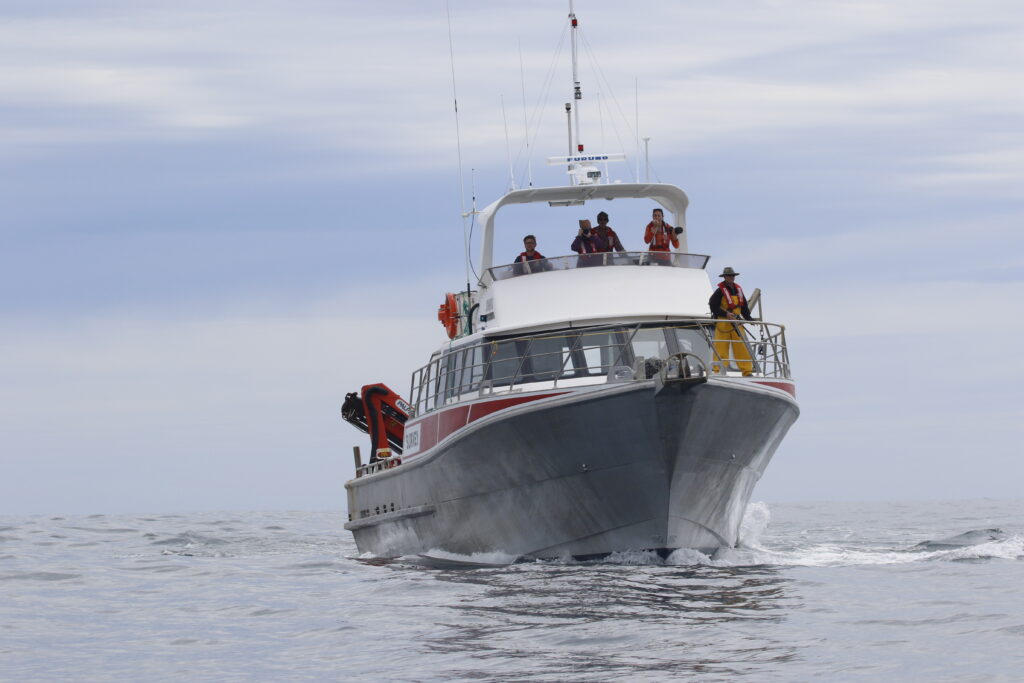
The moving pieces are many as we work to obtain research permits, engage in important consultation with iwi (indigenous Māori groups), procure specialized scientific equipment, and make travel and shipping arrangements. The to-do lists seem to grow just as fast as we can check items off; such is the nature of coordinating an international, multidisciplinary field effort. But it will pay off when we are underway, and I can barely contain my excitement to back on the water with this research team.
Our team has not collected data in the STB since 2017. We know so much more now than we did when studies of this blue whale population were just beginning. For example, we are eager to put our blue whale forecast tool to use, which will hopefully enable us to direct survey effort toward areas of higher blue whale density to maximize data collection. We are keen to see what new insights we gain, and what new questions and challenges arise.
Research team
The SAPPHIRE project will only be possible with the expertise and coordination of the many members of our collaborative group. We are all thrilled to begin this research journey together, and eager to share what we learn.
Principal Investigators:
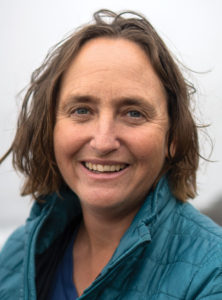
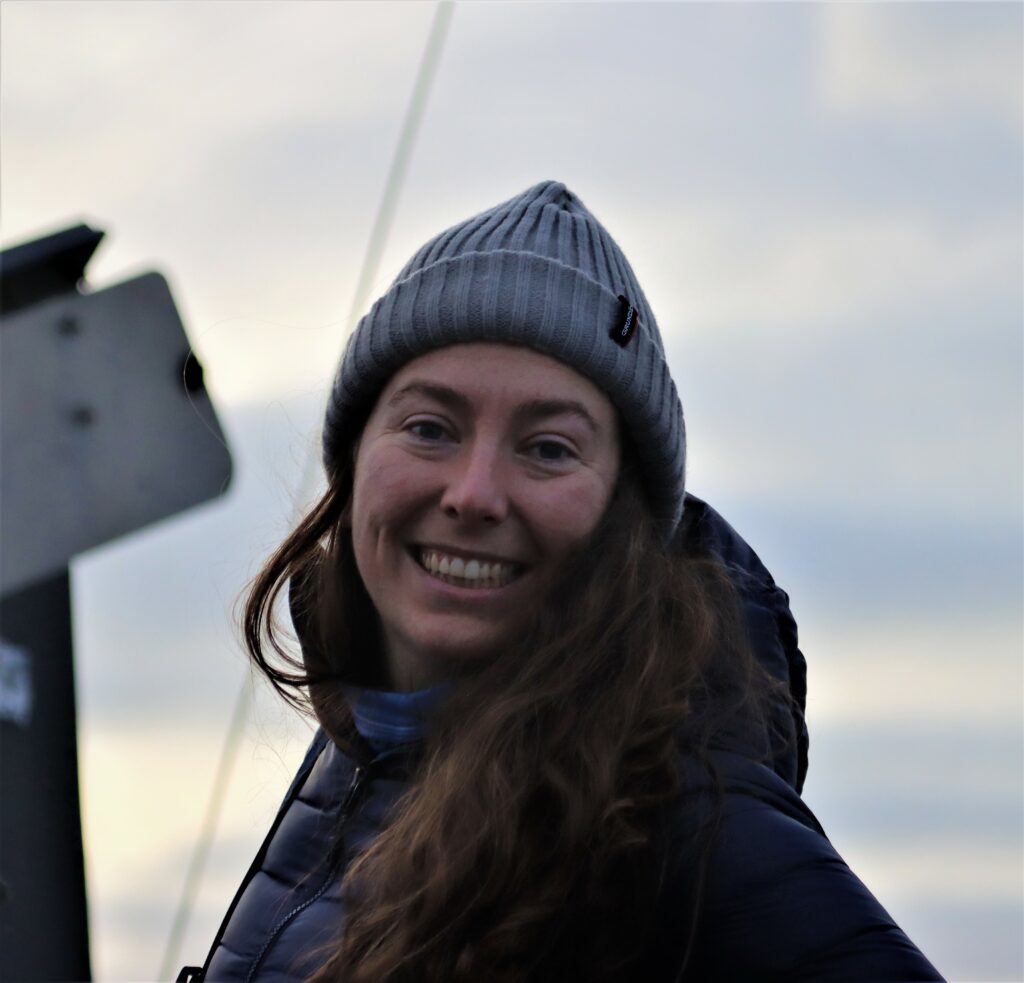
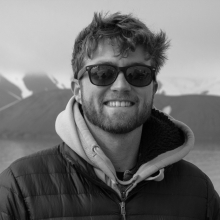
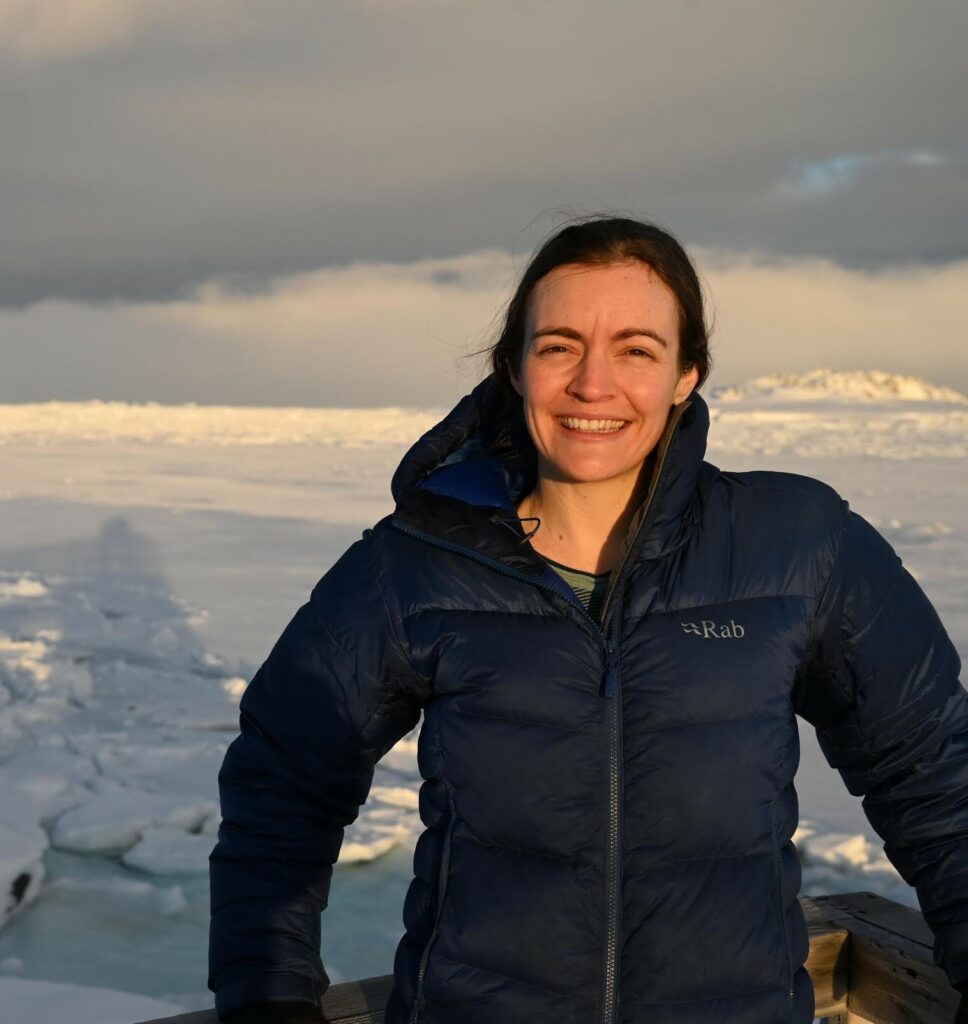
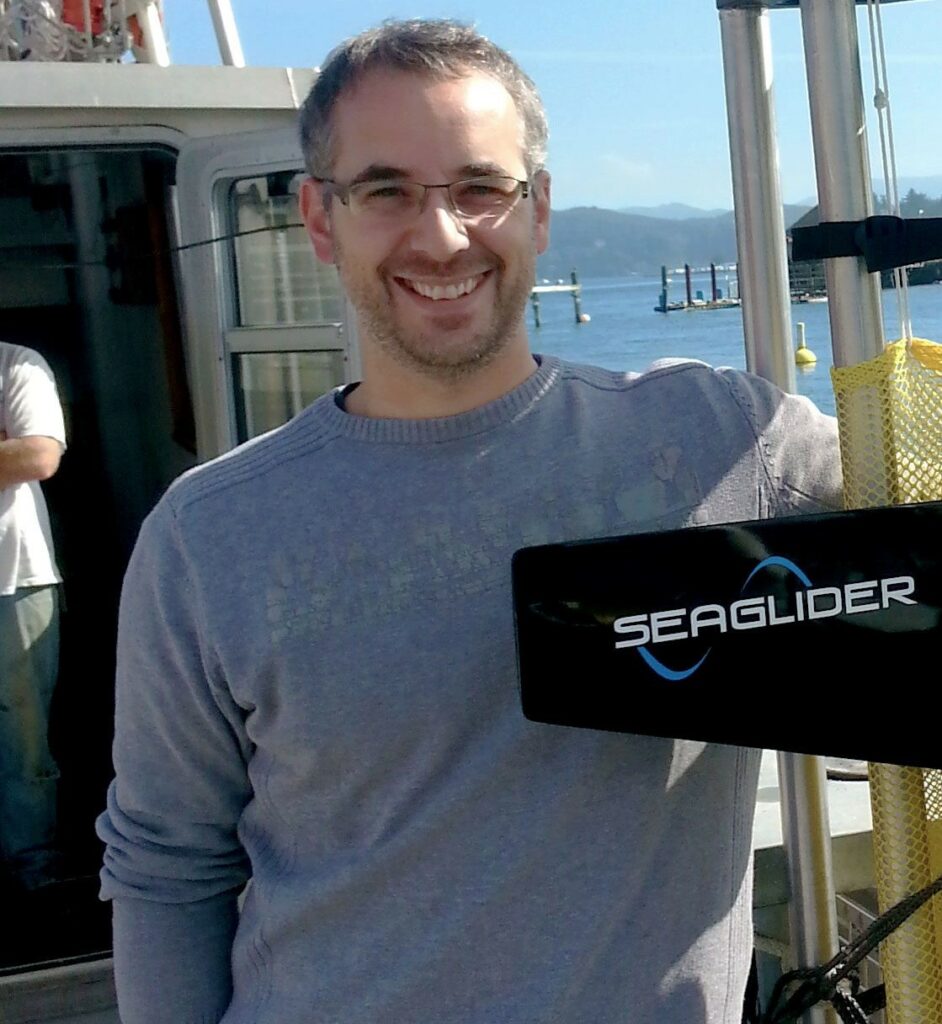
Research partners and key collaborators:
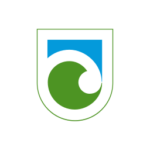
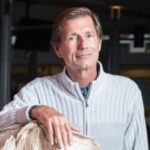
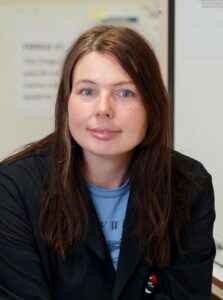
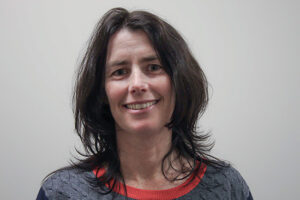
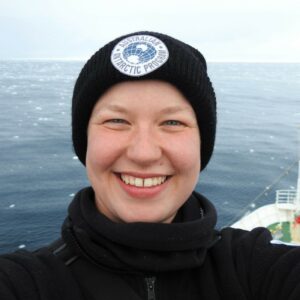
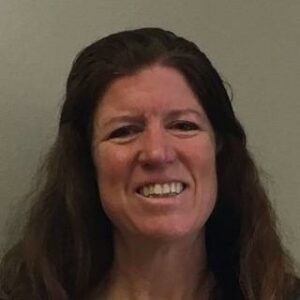
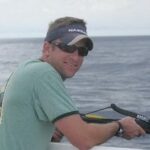
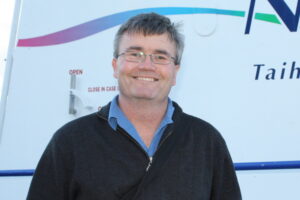
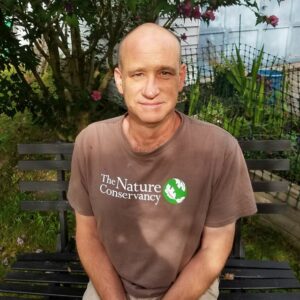
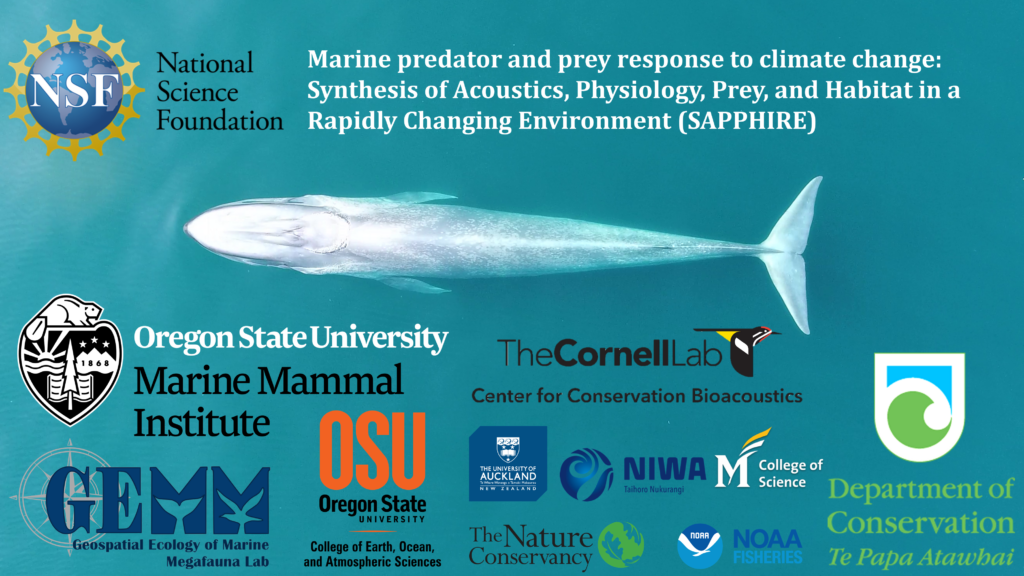
Did you enjoy this blog? Want to learn more about marine life, research, and conservation? Subscribe to our blog and get a weekly alert when we make a new post! Just add your name into the subscribe box below!
References:
1. Barlow DR, Torres LG, Hodge KB, Steel D, Baker CS, Chandler TE, Bott N, Constantine R, Double MC, Gill P, Glasgow D, Hamner RM, Lilley C, Ogle M, Olson PA, Peters C, Stockin KA, Tessaglia-Hymes CT, Klinck H. Documentation of a New Zealand blue whale population based on multiple lines of evidence. Endanger Species Res. 2018;36:27–40.
2. Barlow DR, Klinck H, Ponirakis D, Holt Colberg M, Torres LG. Temporal occurrence of three blue whale populations in New Zealand waters from passive acoustic monitoring. J Mammal. 2022;
3. Barlow DR, Klinck H, Ponirakis D, Branch TA, Torres LG. Environmental conditions and marine heatwaves influence blue whale foraging and reproductive effort. Ecol Evol. 2023;13:e9770.
4. Barlow DR, Klinck H, Ponirakis D, Garvey C, Torres LG. Temporal and spatial lags between wind, coastal upwelling, and blue whale occurrence. Sci Rep. 2021;11(6915):1–10.
5. Barlow DR, Bernard KS, Escobar-Flores P, Palacios DM, Torres LG. Links in the trophic chain: Modeling functional relationships between in situ oceanography, krill, and blue whale distribution under different oceanographic regimes. Mar Ecol Prog Ser. 2020;642:207–25.
6. Torres LG, Barlow DR, Chandler TE, Burnett JD. Insight into the kinematics of blue whale surface foraging through drone observations and prey data. PeerJ. 2020;8:e8906.
7. Barlow DR, Torres LG. Planning ahead: Dynamic models forecast blue whale distribution with applications for spatial management. J Appl Ecol. 2021;58(11):2493–504.