By Imogen Lucciano, incoming graduate student, OSU Department of Fisheries, Wildlife and Conservation Sciences, Geospatial Ecology of Marine Megafauna Lab
There are moments in our individual lifetimes that we can define as noteworthy and right now, as I prepare to start my graduate career within the Marine Mammal Institute (MMI) at OSU, I would say this is it for me. As I sit down to write this blog and document how surreal my future adventure is, I simultaneously feel this path is felicitous. After a year of being cooped up due to COVID, time presently seems to be going by at rocket speed. I am moving constantly in through my day to continue running my current life, while simultaneously arranging all that will encompass my new life. And while I answer questions to my 10-year-old daughter who is doing geometry homework in the living room, while hollering “That is not yours!” to the kitchen where the recently adopted feral dog is sticking his entire head under the trash can lid, while arranging our books in a cardboard box at the packing station I set up on the dining room table, I cannot deny a sense of serenity. This moment in my life, becoming a part of the GEMM Lab and MMI, and relocating to Corvallis is great.
This moment’s noteworthiness is emphasized by embarking on probably the most variable-heavy road trip I have planned to date. Since the age of 19, when I left my small mountain town on the Appalachian trail in Pennsylvania, I have transferred locations ~20 times. Due to extensive travel while serving in the Army (various Army trainings and overseas mission deployments), I have bounced around the US and to other countries often. Over time, one becomes acclimated to the hectic nature of this sort of lifestyle, and yet this new adventure holds significance.
So here are the details of the adventure trip that lies ahead: I will drive my 2002 Jeep Grand Cherokee across the country; from Charlottesville, Virginia to Corvallis, Oregon. My projected route will extend 2,822 miles and take ~43 driving hours total. The route will fall within the boundaries of 11 states (see Figure 1.)
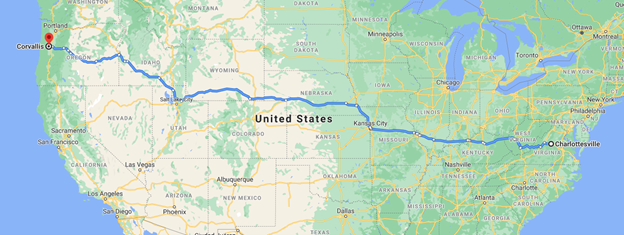
Attached to the hitch of the Jeep will be a 6×12 rented cargo trailer containing our treasured books, furniture and things. Inside the Jeep will be three living variables: Mia (the 10-year-old), Angus (hyperactive border collie/ pit bull mix) and Mr. Gibbs (feral pirate dog); all three will need to be closely monitored for potential hiccups in the plan.
If we are going to make it to our destination hotel/Airbnb each night of the trip, I must be organized and calculate road time each day while factoring in breaks to the loo and fueling up. These calculations need to be precise, with little margin for error. I cannot play it too safely either, or it will take us too long to get across the country (I must start my graduate work after all). On the other hand, I cannot realistically expect too many road hours in a day. I think at this point I have got it worked out (Table 1.)
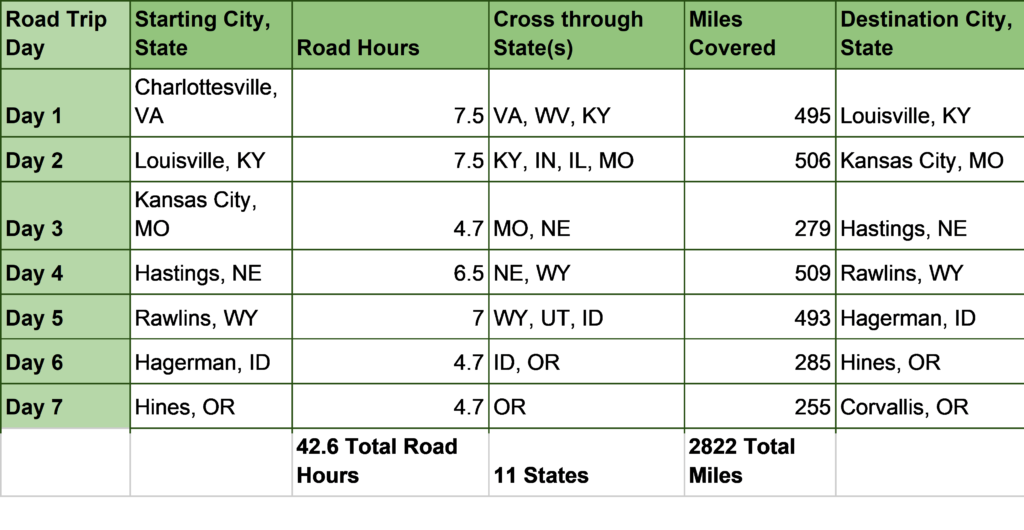
When I look back on my career, I had no idea that my not-so-smooth road would lead me to my dream goal of studying marine mammals. I took the Army placement tests at the age of 19, which led me to the field of “information operations” where I earned a great knowledge base in data analysis and encountered fantastic leaders whom I might not have known otherwise. I learned immensely on this path and it set me up very well for moving forward into research and collaboration in the sciences. I am so grateful that my life took this journey because working in the military provided me with the utmost respect for my opportunities and greater empathy for others. This route had many extreme obstacles and was intensely intimidating at times, but I am all the better for it. And I was never able to shake the dream of where I wanted to be (see Figures 2 & 3.) Timing is everything.


Figure 2 & 3. Two of the images of the Pacific coast I have hung up in my house. Keeping my eye on the prize, so to speak.
It will feel great to cross over the Oregon state line. I cannot wait to meet GEMM Lab in-person and all the other wonderful researchers and staff at MMI and Hatfield Marine Science Center. I am eager to step onto the RV Pacific Storm and begin my thesis research on the magnificent cetaceans off the Oregon coast, and hopefully do some good in the end. As I evaluate the logistics of my trip from Charlottesville to Corvallis, I feel relieved rather than overwhelmed. We could attribute this relief to my not-so-smooth road to get to where I am. Looking ahead, of course, I see a road that will require focus, attention, passion, care, and lots of fuel. Even if this road is not completely smooth, I will have my hands on 10 and 2, and feel so grateful and ready to be on it.