By Rachel Kaplan, PhD student, OSU College of Earth, Ocean and Atmospheric Sciences and Department of Fisheries, Wildlife, & Conservation Sciences, Geospatial Ecology of Marine Megafauna Lab
Krill, a shrimplike crustacean found across our oceans, embodies the term “small but mighty”. Though individuals tend to be small, sometimes weighing in at less than a gram, the numerous species of krill have a global distribution and are estimated to collectively outweigh the entire human population. Much of my graduate research focuses on relationships between foraging whales and krill (Euphausia pacifica and Thysanoessa spinifera) in the Northern California Current (NCC) region. This work hinges on themes that are universal across environments: just as krill are ubiquitous across the global ocean, questions of prey quality, distribution, and ecological relationships with predators are universal.
Next week, I’m headed south to consider these questions in a very different foraging environment: the Western Antarctic Peninsula (WAP). One benefit of being a co-advised student is the incredible opportunity to be exposed to diverse projects and types of research. My graduate co-advisor, Kim Bernard, has studied krill in the WAP region for over a decade, and she is currently leading research into the implications of the shifting polar food web for Antarctic krill (Euphasia superba). Through a series of laboratory experiments and fieldwork, the project, titled “The Omnivore’s Dilemma: The effect of autumn diet on winter physiology and condition of juvenile Antarctic krill”, investigates the impact of climate-driven changes in diet on the health of juvenile krill in autumn and winter, a key time for their survival and recruitment. Winter is a poorly studied season in Antarctica, and this project has already shed light on the physiology, respiration, and growth potential of juvenile krill (Bernard et al., 2022).
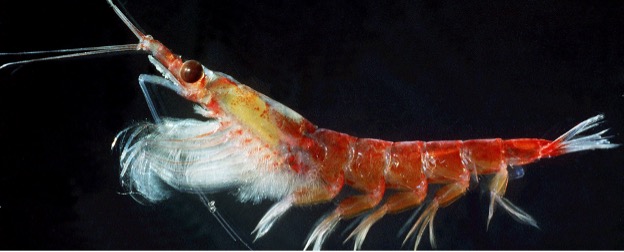
Just as in the NCC region, krill are an essential link in Southern Ocean food webs, where they transfer energy from their microscopic prey to the higher trophic levels that eat them, including several species of fish, seals, penguins, and whales (Bernard & Steinberg, 2013; Cavan et al., 2019; Ducklow et al., 2013). These predators depend upon this high-quality prey to fuel their seasonal migrations and to build the energy reserves they need to survive the frigid Antarctic winter (Cade et al., 2022; Schaafsma et al., 2018). But, the quality of krill depends upon the food that it can consume itself, and climate change may alter their diet.
There’s a lot to love about krill, but my fascination with them is directly tied to their value as a food source for predators. I want to know how the caloric content of individuals and the aggregations they form changes spatially along the WAP, and how this might shift under climate-forced food web changes. This work will clarify the climate-driven variability in the quality of krill as prey, and the implications this might have for top predators in the region.
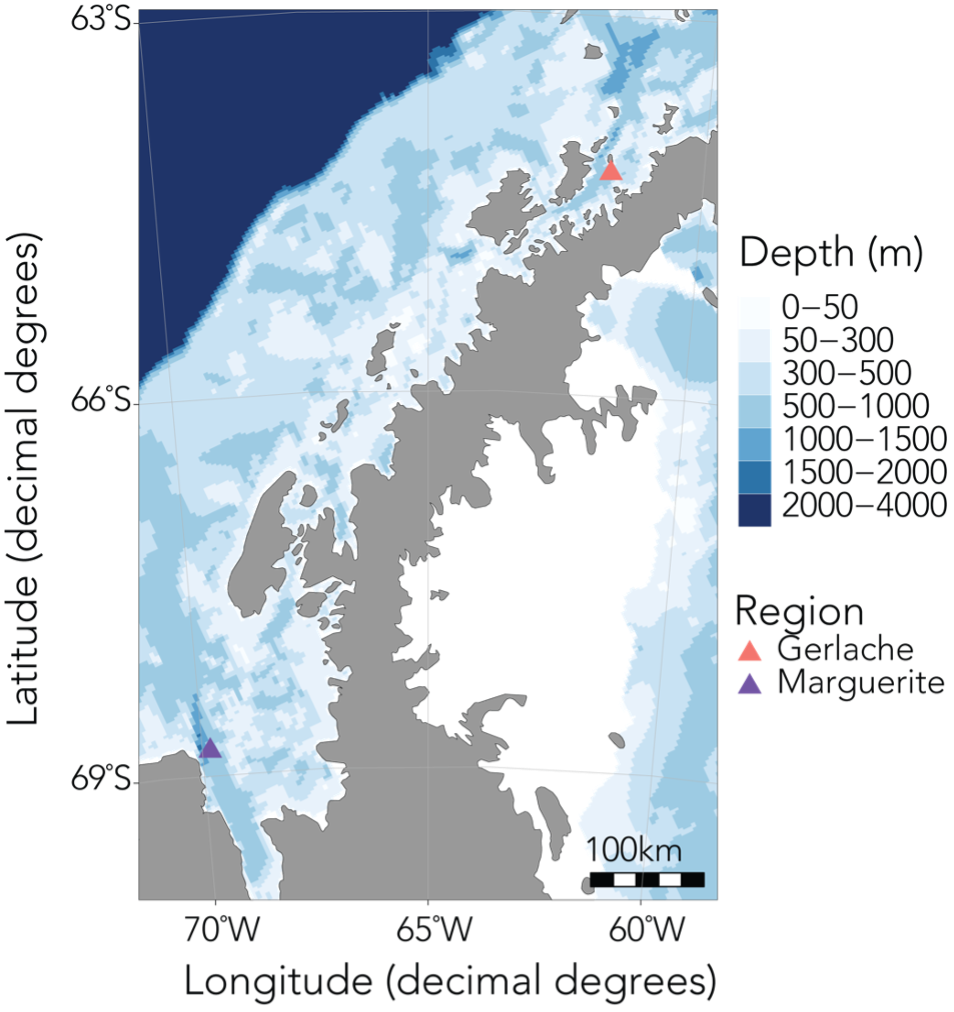
In order to investigate these questions, I’ll be spending the next six months based out of Palmer Station, the smallest of the United States’ research bases in Antarctica, along with Kim and our undergraduate intern Abby. During this upcoming field season, we’ll spend about a month at sea collecting krill samples and active acoustic data using an echosounder, and the rest of the time conducting experiments and sampling in the nearshore. Over the last year, Abby has worked with me to quantify krill caloric content in the NCC, as well as processing samples collected in Antarctica last year. I’m so impressed by everything she’s accomplished, and excited to see her take in this environment, learn a fresh set of experimental and field sampling approaches, and be inspired to ask new questions.
For me, heading south will be a bit like coming home. After graduating from college, I spent about nine months living at Palmer Station and working on the microbial ecology component of the long-term ecological research station there. The experience of being immersed in the WAP environment was foundational to my curiosity about ocean ecology and the impacts of climate change. It is also where I met Kim! All in all, this environment fueled my desire to study krill with Kim and spatial ecology with Leigh, and set me on the course I’m on today.
It also feels meaningful to return here again at this point in my educational journey. With new knowledge and questions I have formed while working in the NCC, I am now excited to apply this knowledge and consider similar questions in the WAP. Abby and I will write blogs through the season and post them here, so stay tuned for news from down south!
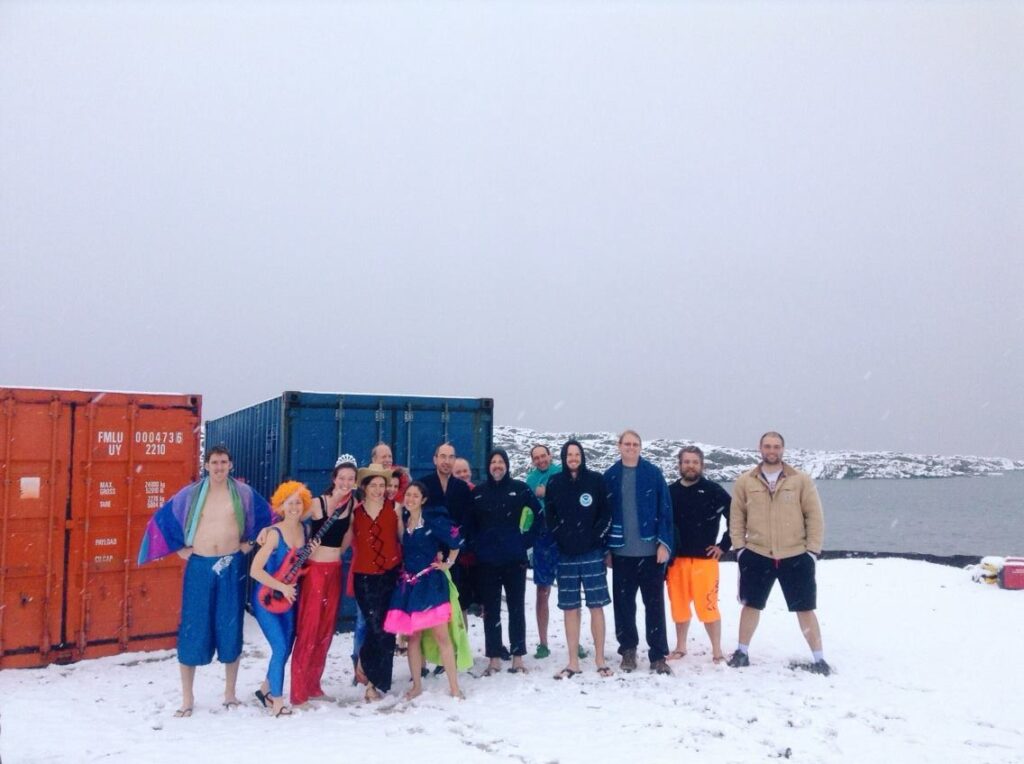
References
Bernard, K. S., & Steinberg, D. K. (2013). Krill biomass and aggregation structure in relation to tidal cycle in a penguin foraging region off the Western Antarctic Peninsula. ICES Journal of Marine Science, 70(4), 834–849. https://doi.org/10.1093/icesjms/fst088
Bernard, K. S., Steinke, K. B., & Fontana, J. M. (2022). Winter condition, physiology, and growth potential of juvenile Antarctic krill. Frontiers in Marine Science, 9, 990853. https://doi.org/10.3389/fmars.2022.990853
Cade, D. E., Kahane-Rapport, S. R., Wallis, B., Goldbogen, J. A., & Friedlaender, A. S. (2022). Evidence for Size-Selective Predation by Antarctic Humpback Whales. Frontiers in Marine Science, 9, 747788. https://doi.org/10.3389/fmars.2022.747788
Cavan, E. L., Belcher, A., Atkinson, A., Hill, S. L., Kawaguchi, S., McCormack, S., Meyer, B., Nicol, S., Ratnarajah, L., Schmidt, K., Steinberg, D. K., Tarling, G. A., & Boyd, P. W. (2019). The importance of Antarctic krill in biogeochemical cycles. Nat Commun, 10(1), 4742. https://doi.org/10.1038/s41467-019-12668-7
Ducklow, H., Fraser, W., Meredith, M., Stammerjohn, S., Doney, S., Martinson, D., Sailley, S., Schofield, O., Steinberg, D., Venables, H., & Amsler, C. (2013). West Antarctic Peninsula: An Ice-Dependent Coastal Marine Ecosystem in Transition. Oceanography, 26(3), 190–203. https://doi.org/10.5670/oceanog.2013.62
Schaafsma, F. L., Cherel, Y., Flores, H., van Franeker, J. A., Lea, M.-A., Raymond, B., & van de Putte, A. P. (2018). Review: The energetic value of zooplankton and nekton species of the Southern Ocean. Marine Biology, 165(8), 129. https://doi.org/10.1007/s00227-018-3386-z