By: Kate Colson, MSc Oceans and Fisheries, University of British Columbia, Institute for the Oceans and Fisheries, Marine Mammal Research Unit
When I wrote my first blog a year ago, I was just starting to dig into the field work and data analysis I had loftily proposed for my graduate degree. Now I am writing to you as a new Master of Science! A little more than a month ago, I successfully defended my thesis research where I used the data from minimally invasive high-resolution accelerometry suction cup tags deployed by the GEMM Lab to estimate the relative energetic cost of different foraging behaviors (see my first blog) of Pacific Coast Feeding Group (PCFG) gray whales that forage during summer months off the coast of Oregon. I learned a lot of new skills through this research project and am excited to share some of my odyssey with you.
To start, I want to highlight the technology that made this work possible: the high-resolution accelerometry suction cup tags. These suction cup tags not only record fine scale data about the whale’s movement and behavior from inertial sensors like accelerometers, magnetometers and gyroscopes, the tags also incorporate video and audio data to record what the whale is seeing and hearing in its environment. I used the data from these tags to achieve two research objectives relating to 1) describing foraging behavior of PCFG gray whales and 2) estimating the relative energetic cost of these behaviors.
Through the hard work of the GEMM Lab field team and collaborators John Calambokidis and Dr. Dave Cade, 10 of these high-resolution accelerometry tags were deployed to collect approximately 91.5 hours of data from PCFG gray whales. Excitingly, two of these tags were deployed on a known mother-daughter pair on the same day. The mother and her 8-year-old daughter were even observed foraging together while tagged later in the day and recorded each other while feeding (Figure 1)!
My first research objective was to quantitatively describe the foraging behaviors of PCFG gray whales. These quantitative descriptions exist for other baleen whale foraging behaviors, such as the lunge feeding behavior of rorqual whales (e.g., humpback, fin, and blue whales) where large mouthfuls of prey are engulfed, or the ram filtration feeding of bowhead and right whales where water is filtered for prey as the whale swims along with its mouth open. High-resolution accelerometry tag data has found that a strong acceleration signal is useful for detecting lunges (Goldbogen et al., 2013) and continuous fluking paired with low swim speeds signal the occurrence of ram filtration feeding (Simon et al., 2009). However, gray whales are the only baleen whales to use suction feeding behavior where the whale rolls to one side and sucks up water to filter for prey. Beyond describing side preferences when performing suction feeding (Woodward & Winn, 2006), this unique foraging behavior of gray whales lacks quantitative descriptions. My thesis works to add quantitative descriptions of gray whale suction feeding to the existing descriptions of baleen whale foraging behavior using high-resolution tag data. Based on previous drone focal follows that qualitatively describe gray whale foraging behaviors (Torres et al., 2018), I hypothesized that body position variables would be important for quantitatively describing PCFG gray whale foraging tactics using tag data. I anticipated that the signals of gray whale suction feeding behavior would be different from the signals of lunge and ram filtration feeding performed by other species of baleen whales (Figure 2).
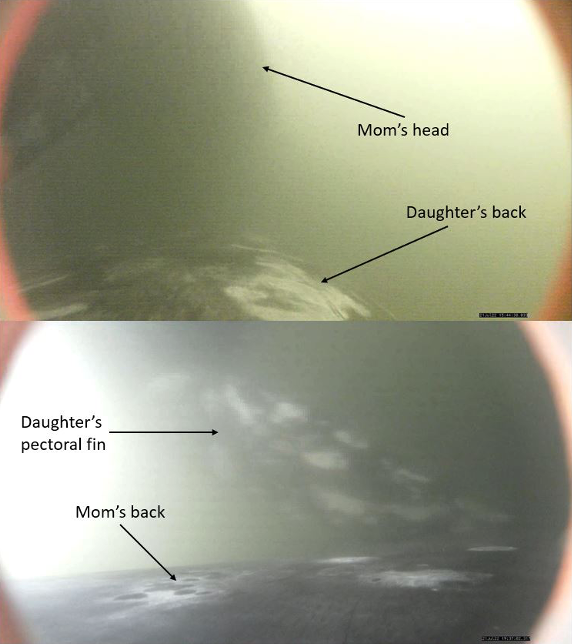
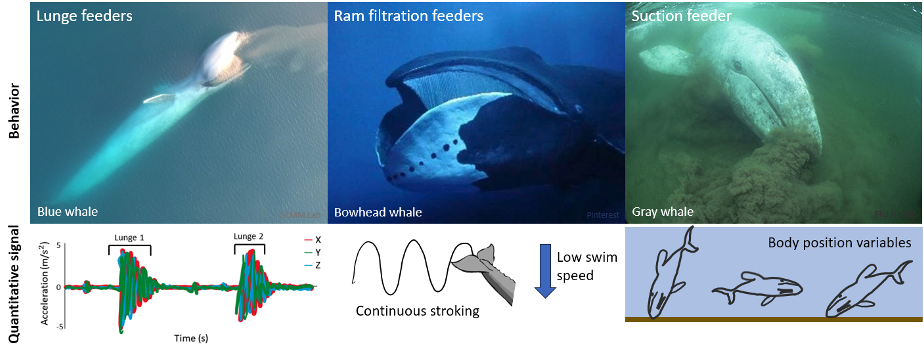
My second objective was to estimate the relative energetic cost of PCFG gray whale foraging behaviors. Previous research has estimated the energetic cost of gray whale broad state behaviors (e.g., transit, search, and forage) using respiration rates (Sumich, 1986). However, respiration rates are difficult to use when estimating the energetic cost of fine scale behaviors, like the precise foraging tactics performed within a dive. Therefore, I calculated biologging-derived proxies of energy expenditure from the tag data such as stroke rate (i.e., the frequency of the whale’s fluke beats) and Overall Dynamic Body Acceleration (ODBA; i.e., the total body movement of the whale) to estimate the relative energetic cost of three different PCFG gray whale foraging tactics: benthic dig, headstand, and side swim. To put these energy expenditure proxies into a human example, if you were walking, your stroke rate would be the frequency of your steps and your ODBA would be all the acceleration of your body including your legs moving with each step, your swinging arms, and turning head. Stroke rate and ODBA are common proxies of energy expenditure that are easily calculated from biologging tag data and have been linked to metabolic rate in many species, including bottlenose dolphins (Allen et al., 2022) and fur seals (Jeanniard-Du-Dot et al., 2016).
When comparing these two proxies of energy expenditure between PCFG gray whale foraging tactics, I expected that the benthic dig foraging behavior would be the least energetically costly compared to the other PCFG foraging tactics. Benthic digs occur when the whale is rolled onto its side and plows through the sediment to suction up prey while leaving feeding pits on the seafloor. Benthic digs are assumed to be the primary foraging tactic of the majority of the gray whale population (Nerini, 1984) that feed in the Arctic and sub-Arctic region where gray whales must dive deeper to reach their prey in the bottom sediments, making it likely that a lower energetic cost of foraging motivates the higher use of this foraging tactic. Excitingly, preliminary results indicate that these three gray whale foraging tactics have different energy expenditures, which can potentially help explain patterns of behavior choice and tactic usage between different groups of gray whales. My thesis research is a foundational step toward better understanding gray whale foraging energetics by providing a means to assess prey requirements and inform management policies to reduce threats facing the PCFG gray whales. For example, previous work has shown that vessel disturbance reduces the searching time (Sullivan & Torres, 2018) and increases the stress levels (Lemos et al., 2022) of PCFG gray whales. My work builds on this by demonstrating an elevated energetic cost of some foraging tactics that could be used to support increasing the distance requirements between vessels and feeding PCFG whales as a way to reduce the energetic impact of vessel disturbance (Figure 3). Additionally, differences in energetic cost of foraging behaviors may help inform potential risks posed by changes in prey quality and quantity for gray whales using different foraging tactics.
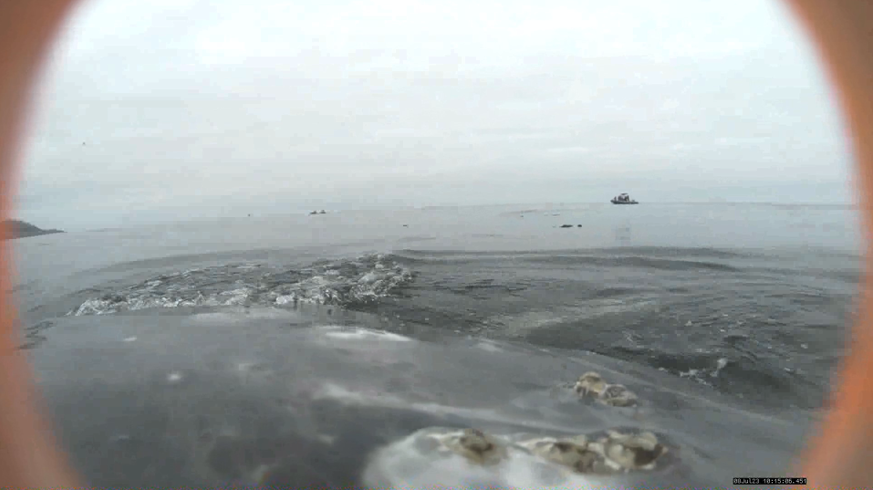
Overall, I am so grateful for my Master’s experience. I had the opportunity to work with amazing scientists that taught me many valuable skills and lessons that I will take with me as I move onto the next phase of my career. Throughout my degree I’ve had a lot of “firsts” and I am excited to embark on another as I prepare my first manuscripts for publication!
References:
Allen, A. S., Read, A. J., Shorter, K. A., Gabaldon, J., Blawas, A. M., Rocho-Levine, J., & Fahlman, A. (2022). Dynamic body acceleration as a proxy to predict the cost of locomotion in bottlenose dolphins. Journal of Experimental Biology, 225(4). https://doi.org/10.1242/jeb.243121
Goldbogen, J. A., Friedlaender, A. S., Calambokidis, J., McKenna, M. F., Simon, M., & Nowacek, D. P. (2013). Integrative approaches to the study of baleen whale diving behavior, feeding performance, and foraging ecology. BioScience, 63(2), 90–100. https://doi.org/10.1525/bio.2013.63.2.5
Izadi, S., Aguilar de Soto, N., Constantine, R., & Johnson, M. (2022). Feeding tactics of resident Bryde’s whales in New Zealand. Marine Mammal Science, 1–14. https://doi.org/10.1111/mms.12918
Jeanniard-Du-Dot, T., Trites, A. W., Arnould, J. P. Y., Speakman, J. R., & Guinet, C. (2016). Flipper strokes can predict energy expenditure and locomotion costs in free-ranging northern and Antarctic fur seals. Scientific Reports, 6. https://doi.org/10.1038/srep33912
Lemos, L. S., Haxel, J. H., Olsen, A., Burnett, J. D., Smith, A., Chandler, T. E., Nieukirk, S. L., Larson, S. E., Hunt, K. E., & Torres, L. G. (2022). Effects of vessel traffic and ocean noise on gray whale stress hormones. Scientific Reports, 12(1). https://doi.org/10.1038/s41598-022-14510-5
Nerini, M. (1984). A review of gray whale feeding ecology. In M. Lou Jones, S. L. Swartz, & S. Leatherwood (Eds.), The gray whale: Eschrichtius robustus (pp. 423–450). Academic Press. https://doi.org/10.1016/B978-0-08-092372-7.50024-8
Simon, M., Johnson, M., Tyack, P., & Madsen, P. T. (2009). Behaviour and kinematics of continuous ram filtration in bowhead whales (Balaena mysticetus). Proceedings of the Royal Society B: Biological Sciences, 276(1674), 3819–3828. https://doi.org/10.1098/rspb.2009.1135
Sullivan, F. A., & Torres, L. G. (2018). Assessment of vessel disturbance to gray whales to inform sustainable ecotourism. Journal of Wildlife Management, 82(5), 896–905. https://doi.org/10.1002/jwmg.21462
Sumich, J. L. (1986). Latitudinal distribution, calf growth and metabolism, and reproductive energetics of gray whales, Eschrichtius robustus. Oregon State University.
Torres, L. G., Nieukirk, S. L., Lemos, L., & Chandler, T. E. (2018). Drone up! Quantifying whale behavior from a new perspective improves observational capacity. Frontiers in Marine Science, 5. https://doi.org/10.3389/fmars.2018.00319
Woodward, B. L., & Winn, J. P. (2006). Apparent lateralized behavior in gray whales feeding off the Central British Columbia coast. Marine Mammal Science, 22(1), 64–73. https://doi.org/10.1111/j.1748-7692.2006.00006.x