By Dawn Barlow, PhD student, OSU Department of Fisheries and Wildlife, Geospatial Ecology of Marine Megafauna Lab
The ocean is vast, ever-changing, and at first glance, seemingly featureless. Yet, we know that the warm, blue tropics differ from icy polar waters, and that temperate kelp forests are different from coral reefs. In the connected fluid environment of the global oceans, how do such different habitats exist, and what separates them? On a smaller scale, you may observe a current mixing line at the ocean surface, or dive down from the surface and feel the temperature drop sharply. In a featureless ocean, what boundaries exist, and how can we delineate between different environments?
These questions have been on my mind recently as I study for my PhD Qualifying Exams, an academic milestone that involves written and oral exams prepared by each committee member for the student. The subject matter spans many different areas, including ecological theory, underwater acoustics, oceanography, zooplankton dynamics, climate change and marine heatwaves, and protected area design. Yet, in my recent studying, I was struck by a realization: since when did my PhD involve so much physics? Atmospheric pressure differences generate wind, which drive global ocean circulation patterns. Density properties of seawater create structure in the ocean, and these physical features influence productivity and aggregate prey for predators such as whales. Sound propagates through the fluid ocean as a pressure wave, and its transmission is influenced by physical characteristics of the sound and the medium it moves through. Many of these examples can be distilled and described with equations rooted in physics. Physics doesn’t behave, it simply… is. In considering the vast and dynamic ocean, there is something quite satisfying in that simple notion.
Circling back to boundaries in the ocean, there are changes in physical properties of the oceans that create boundaries, some stark and some nuanced. These physical features structure and partition the marine environment through differences in properties such as temperature, salinity, density, and pressure. Geographic partitions can occur in both horizontal and vertical dimensions of the water column, and on scales ranging from less than a kilometer to thousands of kilometers [1,2].
In the horizontal dimension, currents, fronts, and eddies mark transition zones between environments. In the time of industrial whaling, observations of temperature and salinity were made at the surface from factory whaling ships and examined to understand where the most whales were available for hunting. These early measurements identified temperature contour lines, or isotherms, and led to observations that whales were found in areas of stark temperature change and places where isotherms bent into “tongues” of interacting water masses [3,4] (Fig. 1). These areas where water masses of different properties meet are often areas of high productivity. Today, we understand that shelf break fronts, river plumes, tidal fronts, and eddies are important horizontal structures that drive elevated nutrient availability, phytoplankton production, and prey availability for mobile marine predators, including whales.
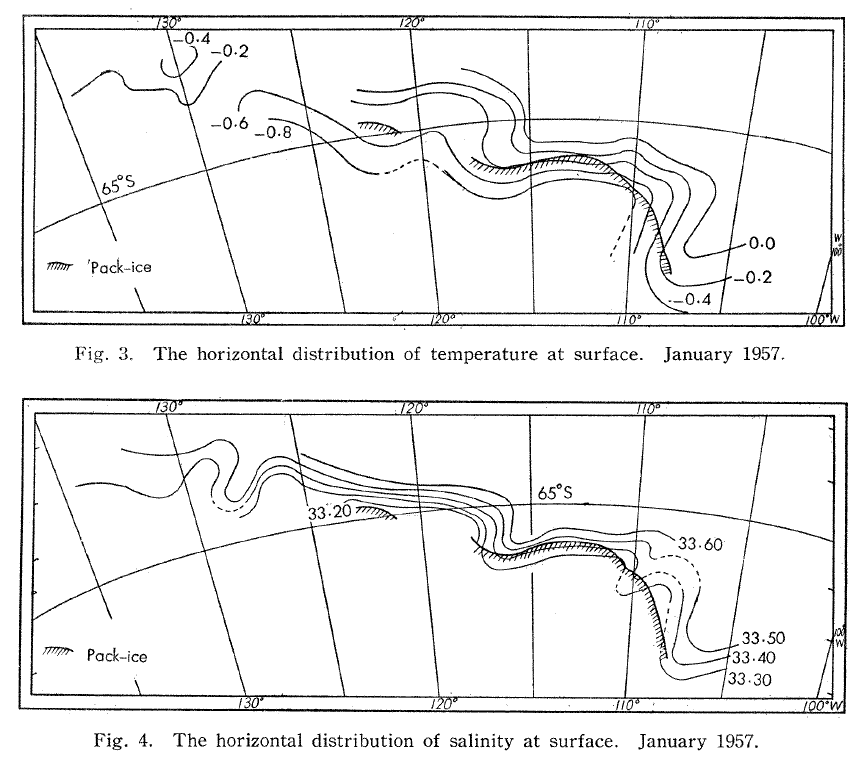
In the vertical dimension, the water column is also structured into distinct layers. Surface waters are warmed by the sunlight and are often lower in salinity due to freshwater input from rain and runoff. Below this distinct surface portion of the water column, the temperature drops sharply in a layer known as the thermocline, and below which pressure and density increase with depth. The surface layer is subject to mixing from wind input, which can draw nutrients from below up into the photic zone and spur productivity. The alternation between stratification—a water column with distinctive layers—and mixing drives optimal conditions for entire food webs to thrive [1,2].
While I began this blog post by writing about boundaries that partition different ocean environments, I have continued to learn that those boundary zones are often critically important in their own right. I started by thinking about boundaries in terms of their importance for separation, but now understand that the leaky points between them actually spur ocean productivity. Features such as fronts, currents, mixed layers, and eddies separate water masses of different properties. However, they are not truly complete and rigid boundaries, and precisely for that reason they are uniquely important in promoting productive marine ecosystems.
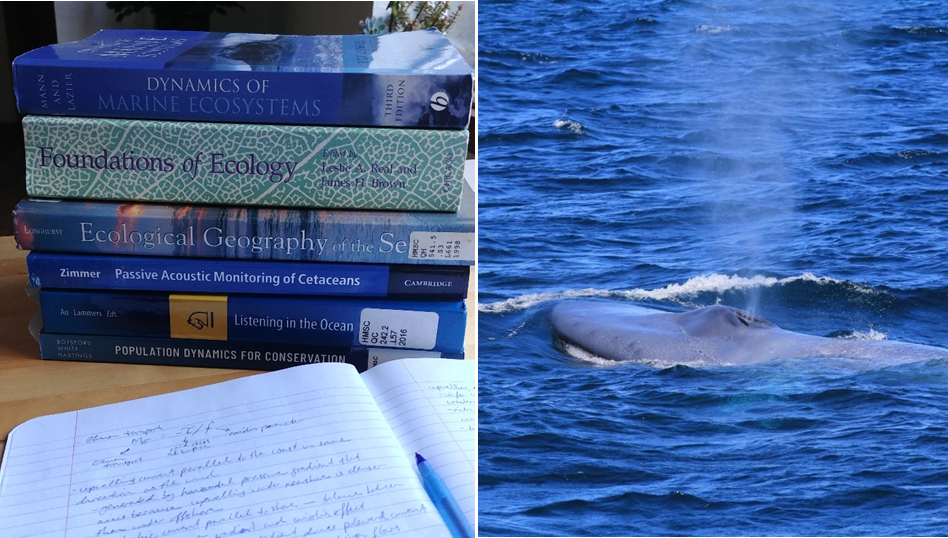
Many thanks to my PhD Committee members who continue to guide me through this degree and who I am lucky to learn from. In particular, the contents of this blog post were inspired by materials recommended by, and discussions with, Dr. Daniel Palacios.
References:
1. Mann, K.H., and Lazier, J.R.N. (2006). Dynamics of Marine Ecosystems 3rd ed. (Blackwell Publishing).
2. Longhurst, A.R. (2007). Ecological Geography of the Sea 2nd ed. (Academic Press).
3. Nasu, K. (1959). Surface water conditions in the Antarctic whaling pacific area in 1956-57.
4. Machida, S. (1974). Surface temperature fields in the Crozet and Kerguelen whaling grounds. Sci. Reports Whales Res. Inst. 26, 271–287.