By Rachel Kaplan, PhD candidate, Oregon State University College of Earth, Ocean, and Atmospheric Sciences and Department of Fisheries, Wildlife, and Conservation Sciences, Geospatial Ecology of Marine Megafauna Lab
The Northern California Current region feeds a taxonomically diverse suite of top predators, including numerous species of seabirds, fish, and marine mammals. Baleen whales such as blue, fin, and humpback whales make this productive area a long stop on their seasonal migration, drawn in large part by abundant krill, a shrimp-like zooplankton that serves as an important prey item.
Aspects of both quality and quantity determine whether a prey resource is advantageous for a predator. In the case of whales, sheer biomass is key. It takes a lot of tiny krill to sustain a large whale – literally tons for a blue whale’s daily diet (Goldbogen et al., 2015). Baleen whales are such big eaters that they actually reshape the ocean ecosystem around them (Savoca et al., 2021).
But the quality of prey, in addition to its quantity, is crucial to energetic profitability, and baleen whales must weigh both elements in their foraging decisions. The outcome of those calculations manifest in the diverse feeding strategies that whales employ across ecosystems. In the California Current region, blue whales preferentially target the larger, more lipid-rich krill Thysanoessa spinifera (Fiedler et al., 1998). In Antarctica, humpback whales target larger and reproductive krill with higher energetic value, if these extra-juicy varieties are available (Cade et al., 2022). Prey-switching, a strategy in which animals target prey based on relative availability, allows fin whales to have a more broad diet than blue whales, which are obligate krill predators.
So, what makes krill of high enough quality for a whale to pursue – or low enough quality to ignore? Krill are widely distributed across the NCC region, so why do foraging whales target one krill patch over another?
That whale of a question combines behavior, foraging theory, biochemistry, physics, climate, and more. One key aspect is the composition of a given prey item. Just as for human diet, nutrients, proteins, and calories are where the rubber hits the road in an animal’s energetic budget. The energy density of prey items sets the cost of living for cetaceans, and shapes the foraging strategies they use (Goldbogen et al., 2015; Spitz et al., 2012). In the NCC, T. spinifera krill are more lipid-rich than Euphausia pacifica (Fisher et al., 2020). Pursuing more energy dense prey increases the profitability of a given mouthful and helps a whale offset the energy expended to earn it, including the costly hunt for prey on the foraging grounds (Videsen et al., 2023).
Krill are amazingly dynamic animals in their own right, and they have evolved life history strategies to accommodate a broad range of ocean conditions. They can even exhibit “negative growth,” shrinking their body length in response to challenging conditions or poor food quality. This plasticity in body size can allow krill to survive lean times – but from the perspective of a hungry whale, this strategy also shrinks the available biomass into smaller packages (Robertson & Bjorkstedt, 2020).
One reason why krill are such advantageous prey type for baleen whales is their tendency to aggregate into dense swarms that may contain hundreds of thousands of individuals. The large body size of baleen whales requires them to feed on such profitable patches (Benoit-Bird, 2024). The packing density of krill within aggregations determines how many a whale can capture in one mouthful, and drives patch selection, such as for blue whales in Antarctica (Miller 2019).
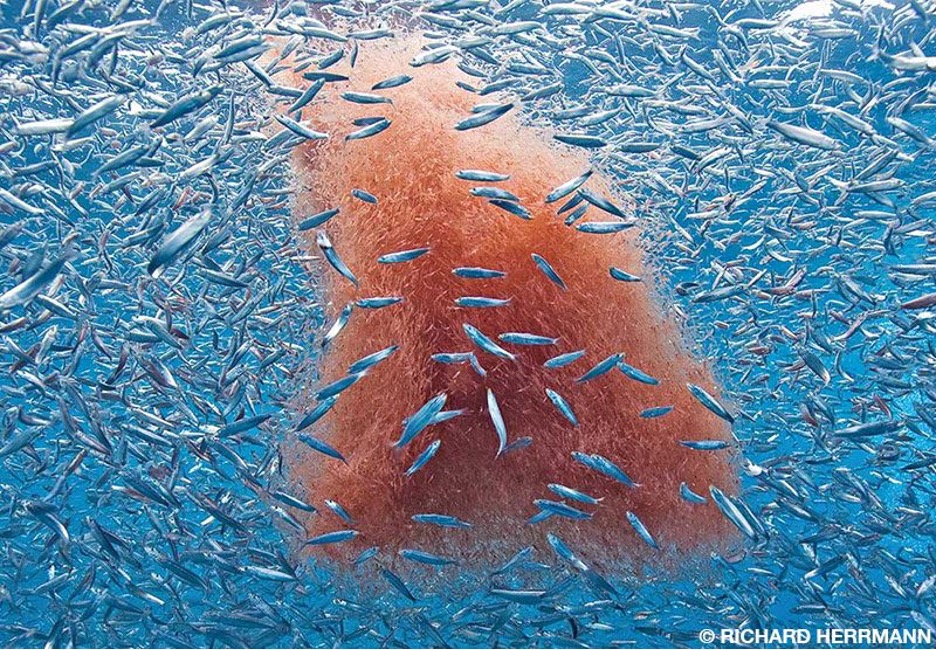
However, even the juiciest, densest krill won’t benefit a foraging whale if the energy required to consume it outweighs the gains. The depth of krill in the water column shapes the acrobatic foraging maneuvers blue whales use to feed (Goldbogen et al., 2015), and is a key driver of patch selection (Miller et al., 2019). The horizontal distance between the whale and a new krill patch is important too. Foraging humpback whales adapt their movements to the hierarchical structure of the preyfield, and feeding on neighboring prey schools can reduce the energy and time expended during interpatch travel, increasing net foraging gain (Kirchner et al., 2018).
Prey quality is dynamic, shaped by environmental conditions, extreme events, and climate change processes (Gomes et al., 2024). We can’t yet fully predict how change will alter prey and predator relationships in the NCC region (Muhling et al., 2020), making every step toward understanding prey dynamics relative to environmental variability key to anticipating how whales will fare in an unknown future (Hildebrand et al., 2021). If you are what you eat, then learning more about krill prey quality will give us unique insights into the baleen whales that come from far and wide to the NCC foraging grounds.
References
Benoit-Bird, K. J. (2024). Resource Patchiness as a Resolution to the Food Paradox in the Sea. The American Naturalist, 203(1), 1–13. https://doi.org/10.1086/727473
Cade, D. E., Kahane-Rapport, S. R., Wallis, B., Goldbogen, J. A., & Friedlaender, A. S. (2022). Evidence for Size-Selective Predation by Antarctic Humpback Whales. Frontiers in Marine Science, 9, 747788. https://doi.org/10.3389/fmars.2022.747788
Fiedler, P. C., Reilly, S. B., Hewitt, R. P., Demer, D., Philbrick, V. A., Smith, S., Armstrong, W., Croll, D. A., Tershy, B. R., & Mate, B. R. (1998). Blue whale habitat and prey in the California Channel Islands. Deep Sea Research Part II: Topical Studies in Oceanography, 45(8–9), 1781–1801. https://doi.org/10.1016/S0967-0645(98)80017-9
Fisher, J. L., Menkel, J., Copeman, L., Shaw, C. T., Feinberg, L. R., & Peterson, W. T. (2020). Comparison of condition metrics and lipid content between Euphausia pacifica and Thysanoessa spinifera in the northern California Current, USA. Progress in Oceanography, 188. https://doi.org/10.1016/j.pocean.2020.102417
Goldbogen, J. A., Hazen, E. L., Friedlaender, A. S., Calambokidis, J., DeRuiter, S. L., Stimpert, A. K., & Southall, B. L. (2015). Prey density and distribution drive the three‐dimensional foraging strategies of the largest filter feeder. Functional Ecology, 29(7), 951–961. https://doi.org/10.1111/1365-2435.12395
Gomes, D. G. E., Ruzicka, J. J., Crozier, L. G., Huff, D. D., Brodeur, R. D., & Stewart, J. D. (2024). Marine heatwaves disrupt ecosystem structure and function via altered food webs and energy flux. Nature Communications, 15(1), 1988. https://doi.org/10.1038/s41467-024-46263-2
Hildebrand, L., Bernard, K. S., & Torres, L. G. (2021). Do Gray Whales Count Calories? Comparing Energetic Values of Gray Whale Prey Across Two Different Feeding Grounds in the Eastern North Pacific. Frontiers in Marine Science, 8. https://doi.org/10.3389/fmars.2021.683634
Kirchner, T., Wiley, D., Hazen, E., Parks, S., Torres, L., & Friedlaender, A. (2018). Hierarchical foraging movement of humpback whales relative to the structure of their prey. Marine Ecology Progress Series, 607, 237–250. https://doi.org/10.3354/meps12789
Miller, E. J., Potts, J. M., Cox, M. J., Miller, B. S., Calderan, S., Leaper, R., Olson, P. A., O’Driscoll, R. L., & Double, M. C. (2019). The characteristics of krill swarms in relation to aggregating Antarctic blue whales. Scientific Reports, 9(1), 16487. https://doi.org/10.1038/s41598-019-52792-4
Muhling, B. A., Brodie, S., Smith, J. A., Tommasi, D., Gaitan, C. F., Hazen, E. L., Jacox, M. G., Auth, T. D., & Brodeur, R. D. (2020). Predictability of Species Distributions Deteriorates Under Novel Environmental Conditions in the California Current System. Frontiers in Marine Science, 7. https://doi.org/10.3389/fmars.2020.00589
Robertson, R. R., & Bjorkstedt, E. P. (2020). Climate-driven variability in Euphausia pacifica size distributions off northern California. Progress in Oceanography, 188. https://doi.org/10.1016/j.pocean.2020.102412
Savoca, M. S., Czapanskiy, M. F., Kahane-Rapport, S. R., Gough, W. T., Fahlbusch, J. A., Bierlich, K. C., Segre, P. S., Di Clemente, J., Penry, G. S., Wiley, D. N., Calambokidis, J., Nowacek, D. P., Johnston, D. W., Pyenson, N. D., Friedlaender, A. S., Hazen, E. L., & Goldbogen, J. A. (2021). Baleen whale prey consumption based on high-resolution foraging measurements. Nature, 599(7883), 85–90. https://doi.org/10.1038/s41586-021-03991-5
Spitz, J., Trites, A. W., Becquet, V., Brind’Amour, A., Cherel, Y., Galois, R., & Ridoux, V. (2012). Cost of Living Dictates what Whales, Dolphins and Porpoises Eat: The Importance of Prey Quality on Predator Foraging Strategies. PLoS ONE, 7(11), e50096. https://doi.org/10.1371/journal.pone.0050096
Videsen, S. K. A., Simon, M., Christiansen, F., Friedlaender, A., Goldbogen, J., Malte, H., Segre, P., Wang, T., Johnson, M., & Madsen, P. T. (2023). Cheap gulp foraging of a giga-predator enables efficient exploitation of sparse prey. Science Advances, 9(25), eade3889. https://doi.org/10.1126/sciadv.ade3889