Getting to the bottom of what top predators in an ecosystem are eating is critical to understand how they may be influencing dynamics in the entire system and food web. But how do you figure out what a predator is eating if it’s hard to catch and collar or watch continuously? Easy, you use their poop! Ellen Dymit, a 4th year graduate student in the Department of Fisheries, Wildlife, and Conservation Sciences advised by Dr. Taal Levi, is our guest on the show this week and she is a poop-tracker extraordinaire!
For her PhD research, Ellen uses primarily non-invasive genetic methods to study large carnivores in two projects in Alaska and Central America. While the systems and carnivores she studies for these two projects are pretty different, the techniques she uses to analyze the collected scats are the same. The Alaska project is focused on determining what different wolf populations and packs across coastal Alaska are consuming, whether individuals are specialized in their feeding habits, and how large the populations are. The Central America project, which is based out of Guatemala, looks at a whole host of predators, including jaguars, pumas, and ocelots, to gain a better understand of the food web dynamics in the ecosystem.
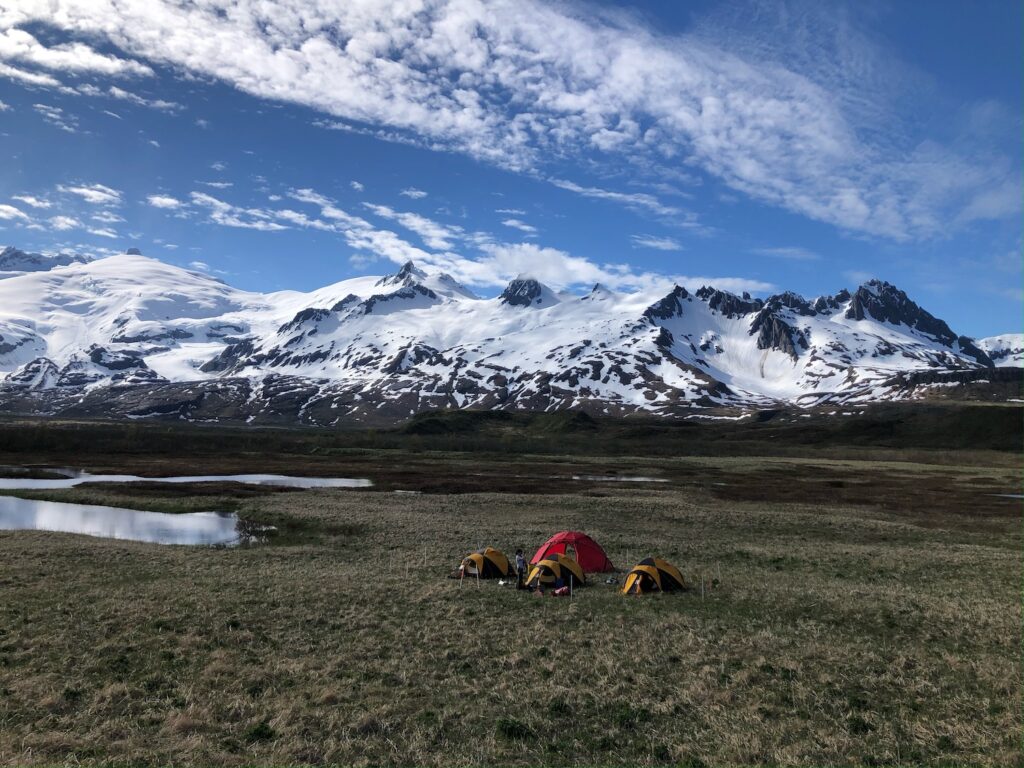
Both of these projects involve some unique challenges in the field that Ellen has had to learn to tackle. DNA can deteriorate pretty quickly, especially in warm Guatemalan temperatures, which is problematic when you’re trying to analyze it. Yet, Ellen’s lab has perfected methods over the last few years to work with neotropical samples. Ellen’s Alaska field work is incredibly remote as it’s just Ellen and one field technician roaming the Alaskan tundra in search of wolf scat. Accessing her field sites involves being flown in on a small fixed wing plane, where they are extremely space and weight-limited. Therefore, every single piece of gear needs to be weighed to ensure that the pilot has enough fuel to get to the site and back. As a result, Ellen isn’t able to collect the entire scat samples that she finds but can only take a small, representative sample.
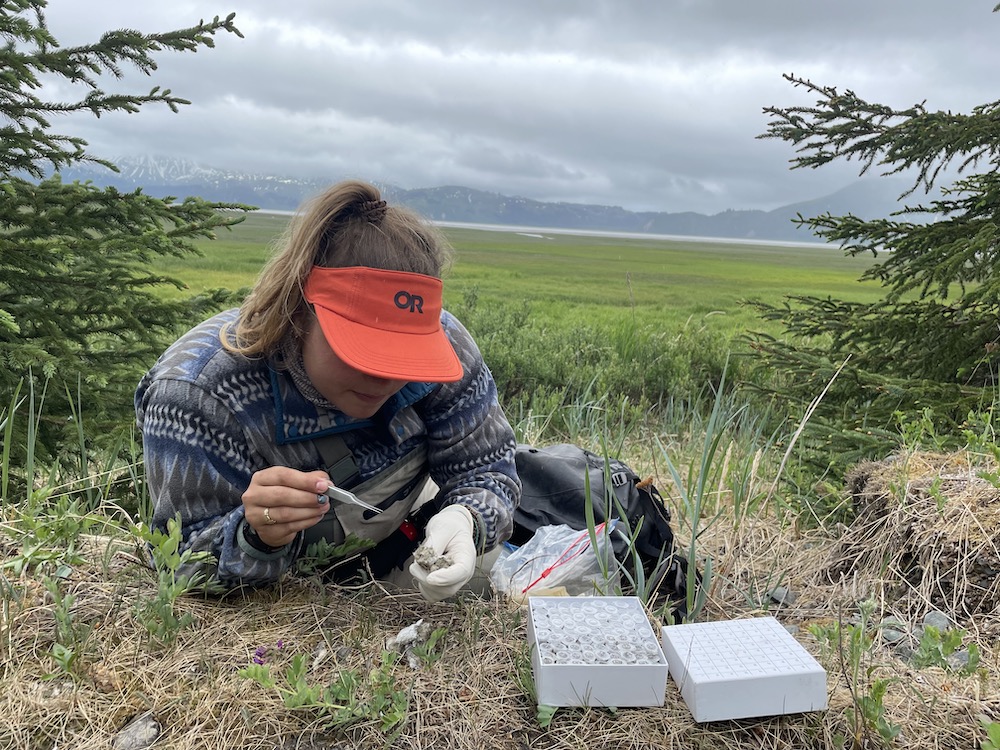
Ellen’s incredibly adventurous field work is followed by months spent in the lab processing her precious scat samples. So far, her results have revealed some pretty interesting differences in diet of wolf packs and populations across three field sites in Alaska. The Guatemalan project, which occurs in collaboration with the Wildlife Conservation Society Guatemala, is one of the first to analyze a large sample size of ocelot scats and the first to attempt DNA metabarcoding of samples collected in the neotropics.
To hear more details about both of these projects, as well as Ellen’s background and some bad-a$$ stories from her Alaskan field work, tune in this Sunday, October 15th live on 88.7 FM or on the live stream. Missed the show? You can listen to the recorded episode on your preferred podcast platform!