Tree rings famously reflect the age of the tree, but they can also encode information about the environmental conditions throughout the organism’s life. A similar principle motivates the study of ice cores – traces of the ancient atmosphere are preserved in the massive ice caps covering Earth’s polar regions.
This Sunday’s guest is Olivia Williams, a graduate student here at Oregon State who is helping to uncover the wealth of climate information harbored by polar ice cores. Olivia is a member of the College of Earth, Ocean and Atmospheric Sciences (CEOAS), where she is advised by Christo Buizert. Their lab uses ice cores to study paleoclimatology and heads the Center for Oldest Ice Exploration (COLDEX), a multi-institution NSF collaboration.
Drilling an ice core in the Arctic or Antarctic is an expensive and labor-intensive process. As a result, once they have been studied by project leads, most American ice core samples are centrally managed by the National Ice Core Lab in Denver, CO and carefully allocated to labs throughout the country. Researchers analyze cross-sections of the larger ice core sample for many geochemical features, including dust records, stable isotopes, and evidence of volcanic eruptions. Determining the historical levels of carbon dioxide, methane, and other greenhouse gases is one application of ice core analysis that yields important insights into climate change.
Olivia’s project focuses on “melt layers”, which are formed by a large-scale melting and refreezing event. The frequency and intensity of melt layers help characterize polar summer temperatures, and specifically the number of days above freezing. Typically, researchers use visual examination or optical instruments to locate layers with relatively smooth and bubble-free ice. However, such methods can fail further down in ice cores, where clathrate ice formed by increased pressure excludes all bubbles. In response, the lab of Jeffrey Severinghaus at the Scripps Institution of Oceanography developed a chemical method to serve as a supplement. This technique extracts noble gases from the core and compares the ratio of the heavier (xenon and krypton) to argon, the lightest noble gas. Since the heavier noble gases are more water-soluble, spikes in the relative concentration of krypton and xenon suggest that a melting event occurred.
During a typical day in the lab, Williams takes samples from the ice core stored at -20 C in a large walk-in freezer and handles the samples in chilled ethanol baths. She particularly focuses on ice cores from Greenland and time periods such as the last interglacial period ~120 thousand years ago and the early Holocene ~12 thousand years ago. Since the OSU lab’s noble gas methodology is novel, Olivia’s work involves a lot of design and troubleshooting the extraction line, which extracts the trapped gases. One time, she even had to commission a scientific glassblower for custom cold traps in the extraction line.
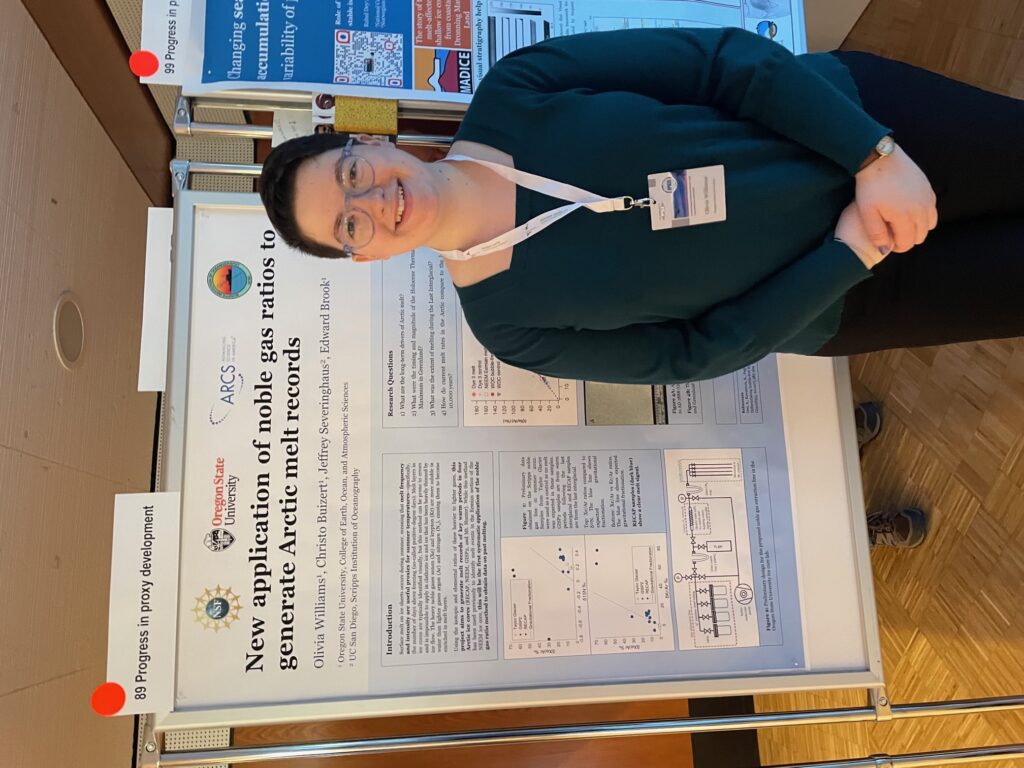
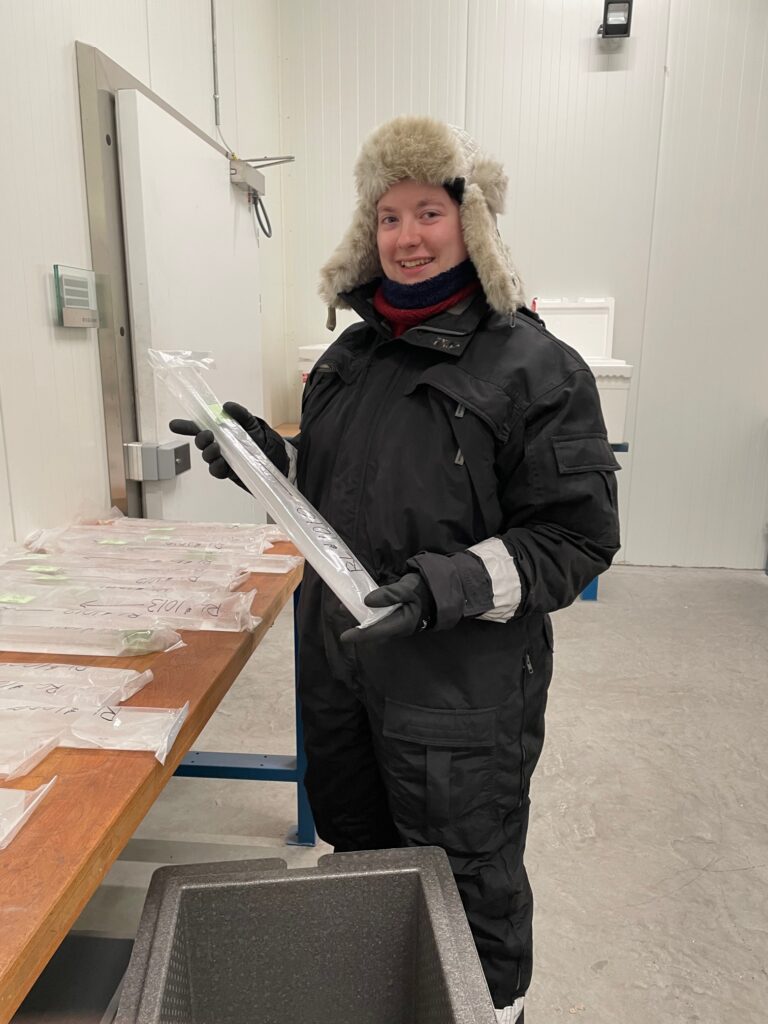
Williams’ interest in geology was impressed upon her at an early age, in part by the influence of her grandfather, a longtime science writer for the Seattle Times. Her grandfather’s love for the geology of the Pacific Northwest inspired her to follow in his footsteps as a scientific journalist. At Boston University, Olivia initially planned to major in communications, until she took a seminar on interdisciplinary science communication offered by BU Antarctic Research Lab, together with education and earth sciences majors. This experience helped solidify her interest in geology, and she switcher her major to earth sciences. Her senior research project related to nutrient cycling in salt marshes, but she knew that she eventually wanted to work in polar science and paleoclimatology. Besides her research at OSU, Olivia has stayed active in science communication, serving as the outreach chair for the CEOS graduate student association. She has helped organize education tables at the Corvallis Farmer’s Market. In the future, Olivia hopes to pursue an academic career and continue research and teaching in the field she loves but is open to the full range of earth science career paths.
For more on Olivia’s exciting research and to hear what it is like to drill ice from a lava formation, tune in this Sunday, January 22nd at 7PM on KBVR 88.7 FM or look out for the podcast upload on Spotify!