David P. Turner / July 16, 2020
Humans are story-telling animals. Our brains are wired to assimilate information in terms of temporal sequences of significant events. We are likewise cultural animals. Within a society, we share images, words, rituals, and stories. Indigenous societies often have myths about their origin and history. Religious mythologies remain prevalent in contemporary societies.
The discipline of Earth System Science has revealed the necessity for a global society that can address emerging planetary scale environmental change issues – notably climate change. A shared narrative about the relationship of humanity to the biosphere, and more broadly to the Earth system, is highly desirable in that context.
The most prevalent narrative about humanity’s relationship to the Earth system emphasizes the growing magnitude of our deleterious impacts on the global environment (think ozone hole, climate change, biodiversity loss). The future of humanity is then portrayed as more of the same, unless radical changes are made in fossil fuel emissions and natural resource management.
In the process of writing a book for use in Global Environmental Change courses, I developed an elaborated narrative for humanity − still based on an Earth system science perspective but somewhat more upbeat. I used the designation Anthropocene Narrative to describe it because Earth system scientists have begun to broadly adopt the term Anthropocene to evoke humanity’s collective impact on the environment.
There are of course many possible narratives evoked by the Anthropocene concept (e.g. the historical role of capitalism in degrading the environment), all worthy of study. But for the purposes of integrating the wide range of material covered in global environmental change classes, I identified a six stage sequence in the relationship of humanity to the rest of the Earth system that serves to link geologic history with human history, and with a speculative vision of humanity’s future (Figure 1). The stages are essentially chapters in the story of humanity’s origin, current challenges, and future. The tone is more hopeful than dystopian because our emerging global society needs a positive model of the future.
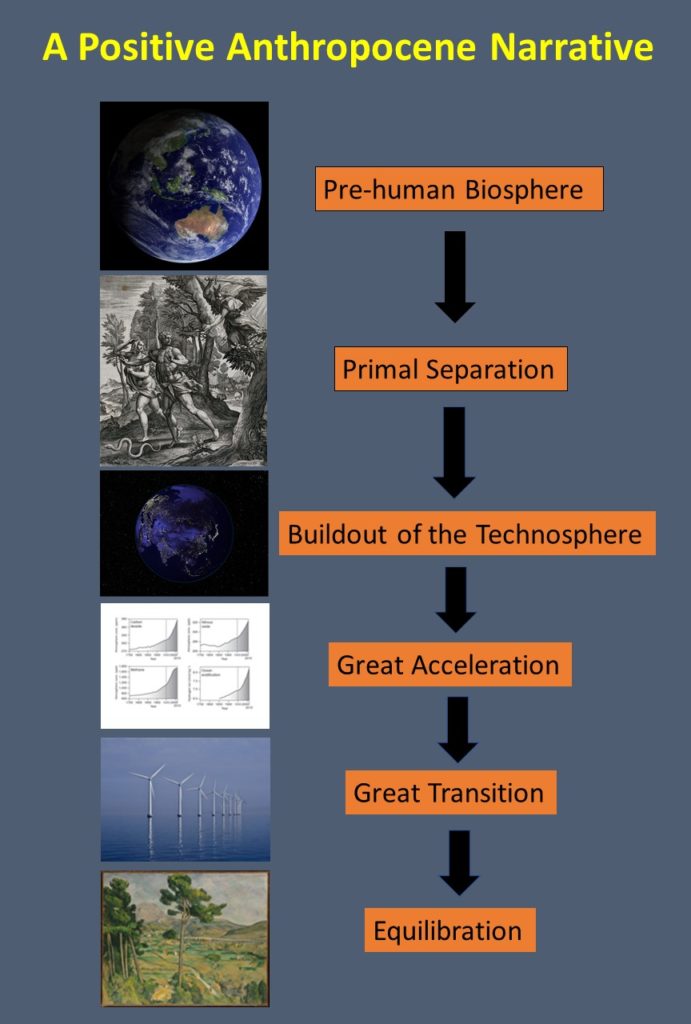
The chapters in this Anthropocene narrative are as follows.
Chapter 1. The Pre-human Biosphere
The biosphere (i.e. the sum of all living organisms) self-organized relatively quickly after the coalescence of Earth as a planet. It is fueled mostly by solar energy. The biosphere drives the global biogeochemical cycles of carbon, nitrogen, and other elements essential to life, and plays a significant role in regulating Earth’s climate, as well as the chemistry of the atmosphere and oceans. The biosphere augments a key geochemical feedback in the Earth system (the rock weathering thermostat) that has helped keep the planet’s climate in the habitable range for 4 billion years. By way of collisions with comets or asteroids, or because of its own internal dynamics, the Earth system occasionally reverts to conditions that are harsh for many life forms (i.e. mass extinction events). Nevertheless, the biosphere has always recovered − by way of biological evolution − and a mammalian primate species recently evolved that is qualitatively different from any previous species.
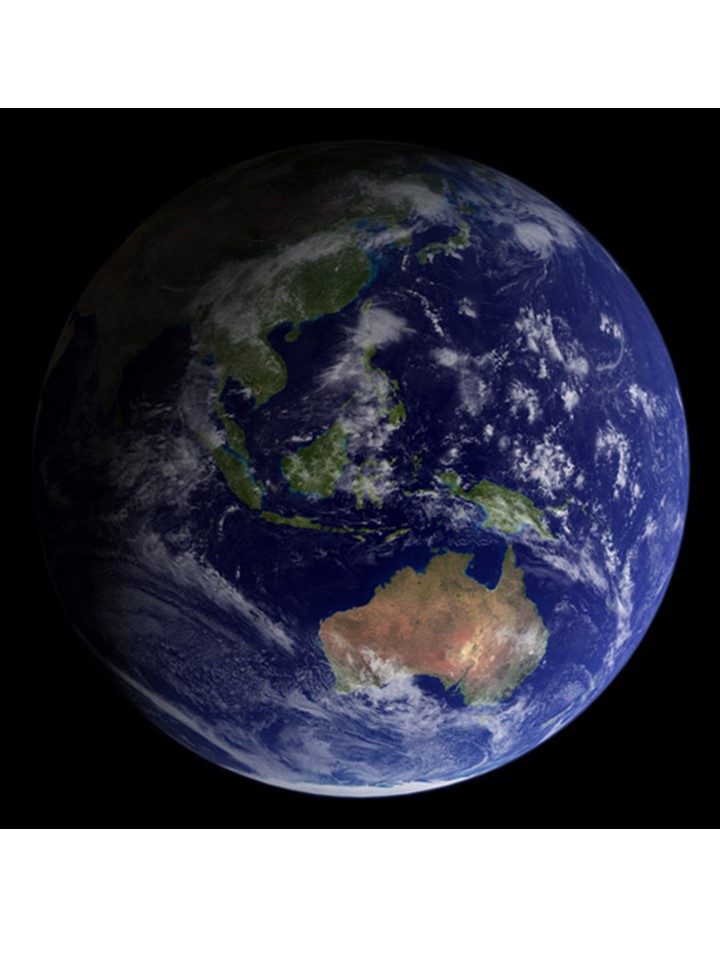
Chapter 2. The Primal Separation
Nervous systems in animals have obvious adaptive significance in term of sensing the environment and coordinating behavior. The brain of a human being appears to be a rather hypertrophied organ of the nervous system that has evolved in support of a capacity for language and self-awareness. These capabilities are quite distinctive among animal species, and they set the stage for human conquest of the planet. The most recent ice age receded about 12,000 year ago and a favorable Holocene climate supported the discovery and expansion of agriculture. With agriculture, and gradual elaboration of toolmaking, humanity ceased waiting for Nature to provide it sustenance. Rather, Nature became an object to be managed. This change is captured in the Christian myth of Adam and Eve’s expulsion from the Garden of Eden (Figure 3). They lived like all other animals in the biosphere until they became self-aware and began to consciously organize their environment.
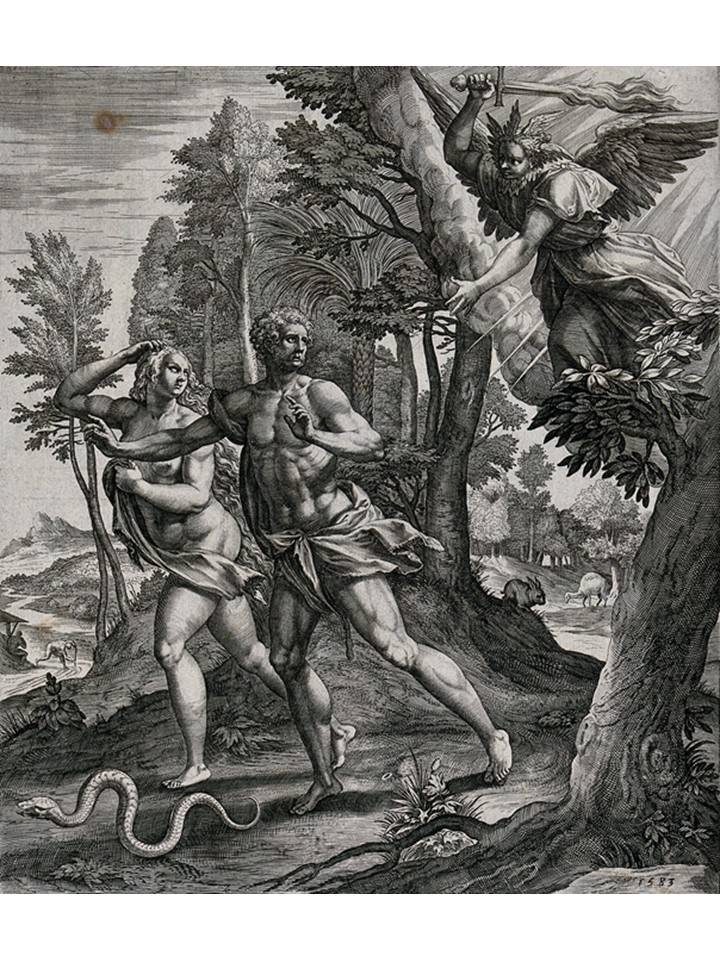
Chapter 3. The Build-out of the Technosphere
The next phase in this narrative is characterized by the gradual evolution and spread of technology. An important driving force was likely cultural group selection, especially with respect to weapons technology and hierarchical social structure. The ascent of the scientific worldview and the global establishment of the market system were key features. Human population rose to the range of billions, and the technosphere began to cloak Earth (Figure 4). The Industrial Revolution vastly increased the rate of energy flow and materials cycling by the human enterprise. Telecommunications and transportation infrastructures expanded, and humanity began to get a sense of itself as a global entity. Evidence that humans could locally overexploit natural resources (e.g. the runs of anadromous salmon in the Pacific Northwest U.S.) began to accumulate.
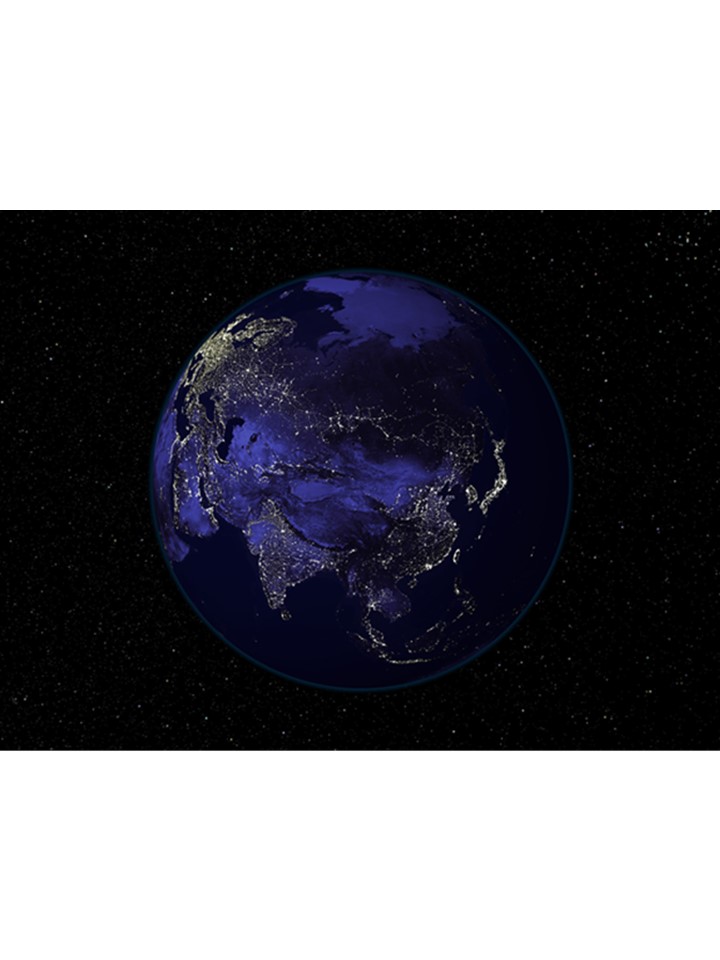
Chapter 4. The Great Acceleration
Between World War II and the present, the global population grew from 2.5 billion to 7.8 billion people. Scientific advances in the medical field reduced human mortality rates and technical advances in agriculture, forestry, and fish harvesting largely kept pace with the growing need for food and fiber. The extent and density of the technosphere increased rapidly. At the same time, we began to see evidence of technosphere impacts on the environment at the global scale – notably changes in atmospheric chemistry (Figure 5) and losses in global biodiversity.
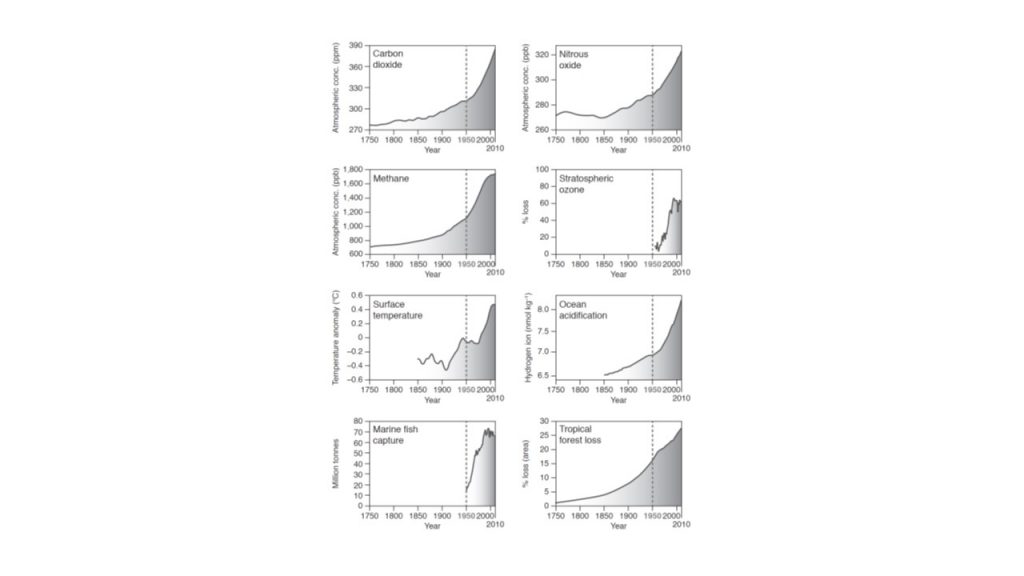
Chapter 5. The Great Transition
This phase is just beginning. Its dominant signal will be the bending of the exponentially rising curves for the Earth system and socio-economic indicators that define the Great Acceleration (Figure 5 above). Global population will peak and decline, along with the atmospheric CO2 concentration. Surviving the aftermath of the Great Acceleration with be challenging, but the Great Transition is envisioned to occur within the framework of a high technology infrastructure (Figure 6) and a healthy global economy. To successfully accomplish this multigenerational task, humanity must begin to function as a global scale collective, capable of self-regulating. Neither hyper-individualism nor populist tribal truth will get us there. It will take psychologically mature global citizens, visionary political leaders, and new institutions for global governance.
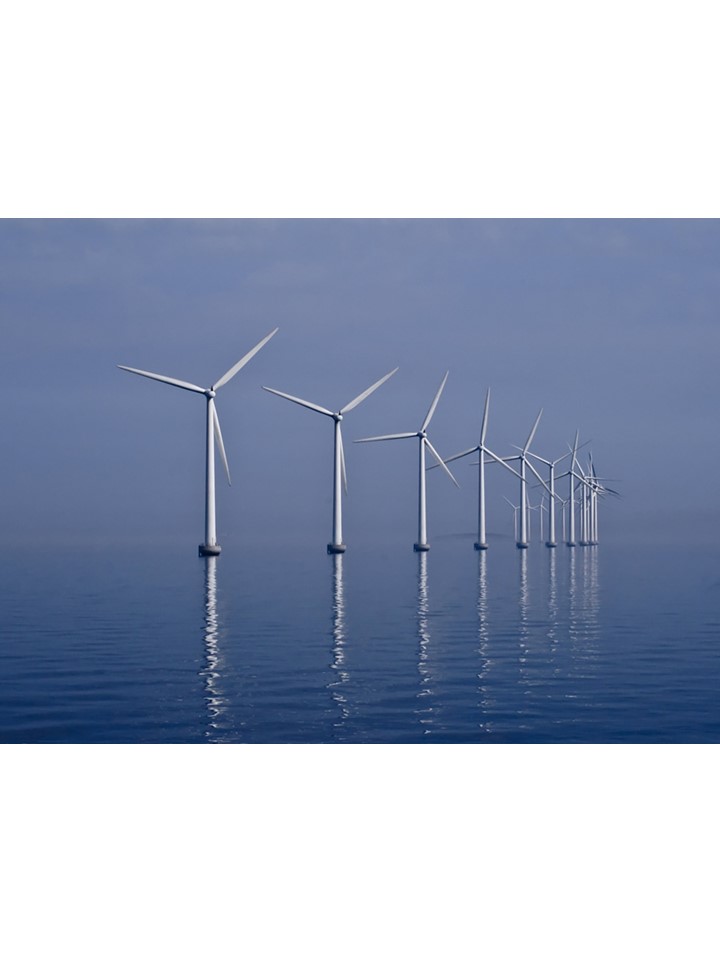
Chapter 6. Equilibration
Human-induced global environmental change will continue for the foreseeable future. The assumption for an Equilibration phase is that humanity will gain sufficient understanding of the Earth system – including the climate subsystem and the global biogeochemical cycles – and develop sufficiently advanced technology to begin using the technosphere and managing the biosphere to purposefully shape the biophysical environment from the scale of ecosystems and landscapes (Figure 7) to the scale of the entire planet. Humanity is a part of the Earth system, meaning it must gain sufficient understanding of the social sciences to produce successive generations of global citizens who value environmental quality and will cooperate to manage and maintain it. The challenges to education will be profound.
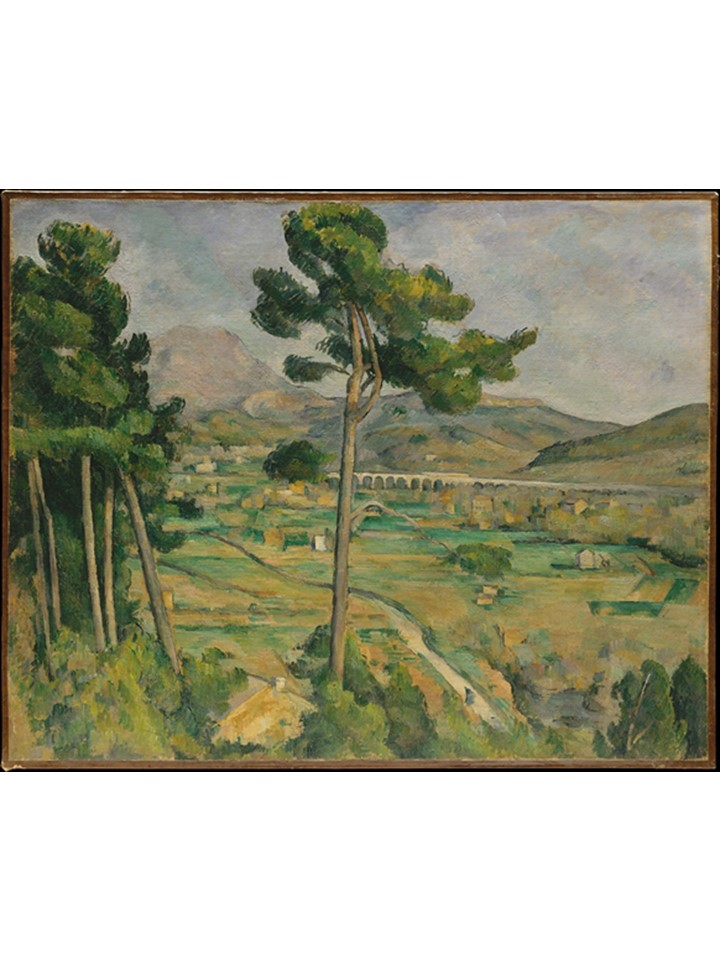
As noted, this Anthropocene Narrative is largely from the perspective of Earth system science. In the interests of coherence, humanity is viewed in aggregate form. Humanities scholars reasonably argue that in the interests of understanding climate justice, “humanity” must be disaggregated (e.g. by geographic region or socioeconomic class). This perspective helps highlight the disproportionate responsibility of the developed world for driving up concentrations of the greenhouse gases. The aggregated and disaggregated perspectives on humanity are complementary; both are needed to understand and address global environmental change issues.
The Anthropocene Narrative developed here is broadly consistent with scientific observations and theories, which gives it a chance for wide acceptance. The forward-looking part is admittedly aspirational; other more dire pathways are possible if not probable. However, this narrative provides a solid rationale for building a global community of all human beings. We are all faced with the challenge of living together on a crowded and rapidly changing planet. The unambiguous arrival of global pandemics and climate change serve as compelling reminders of that fact. A narrative of hope helps frame the process of waking up to the perils and possibilities of our times.
Recommended Video: Welcome to the Anthropocene (~ 3 minutes)
This blog post was featured as a guest blog at the web site for The Millennium Alliance for Humanity and the Biosphere (MAHB).
https://mahb.stanford.edu/blog/a-positive-narrative-for-the-anthropocene/