The Garden Ecology Lab’s Pollinator Plant PR Campaign Presents….. Pearly Everlasting!
The Garden Ecology Lab is releasing a series of plant profiles of the top 10 Oregon native plants for pollinators, based on Aaron Anderson’s 2017-2019 field trials of 23 Oregon native plants. We will feature one plant per week for 10 weeks, this is week 3! Profiles will include photos, planting information, and will highlight common pollinators of each plant.
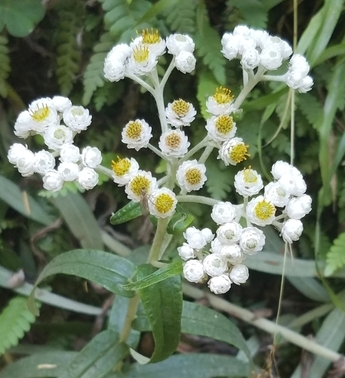
Plant Facts
- Scientific Name: Anaphalis margaritacea
- Life Cycle: Perennial
- Growth Habit: Upright, clumping
- Bloom Duration: June – October
- Hardiness Zone: 3-8
- Special Traits: Drought tolerant, deer resistant
- Light requirements: Prefers full sun but will grow in partial shade
- When to plant: Plant starts in the Spring, or sow seeds directly in the Fall.
Pollinator Facts
- Pearly everlasting was found to be associated with two species of mining bees in Aaron’s research: Andrena cerasifolii, Andrena candida.
- Other common visitors to Pearly Everlasting are American Lady butterflies, Painted Lady butterflies, Everlasting Tebenna moth, and Sweat bees.
- Female and male flowers are generally found on separate Pearly Everlasting plants. This means that male plants provide nectar and pollen to insect visitors while female flowers just provide nectar.
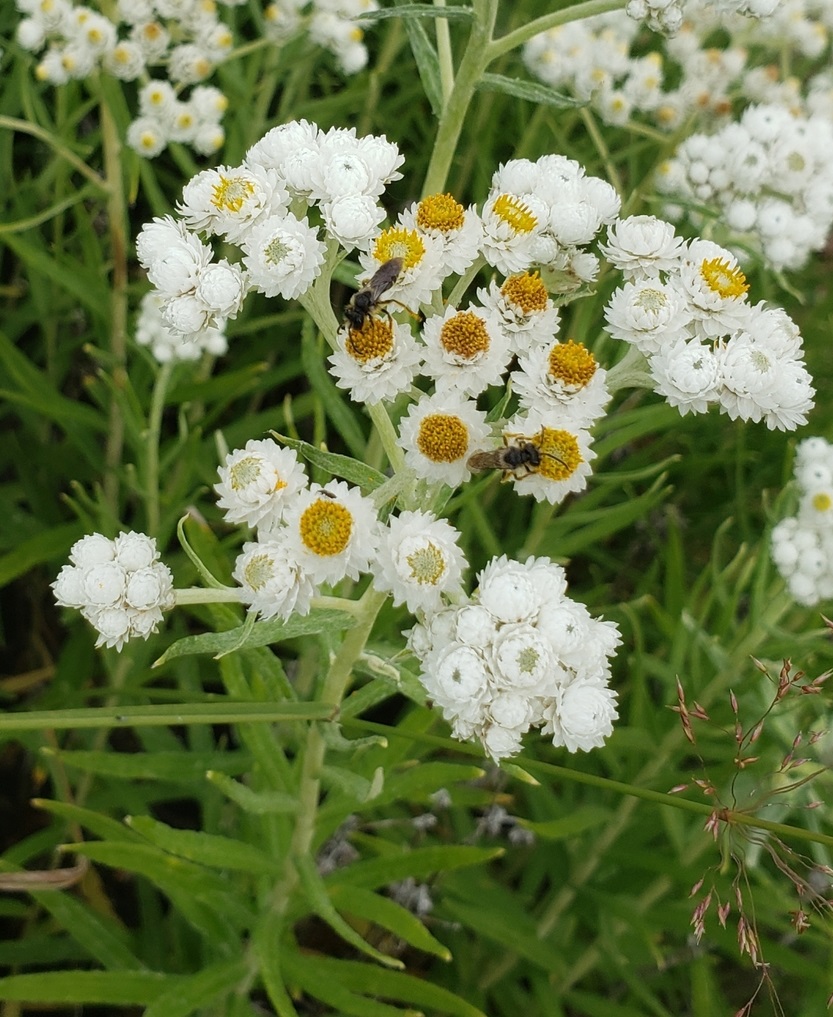
Pearly Everlasting’s Native Range in Oregon
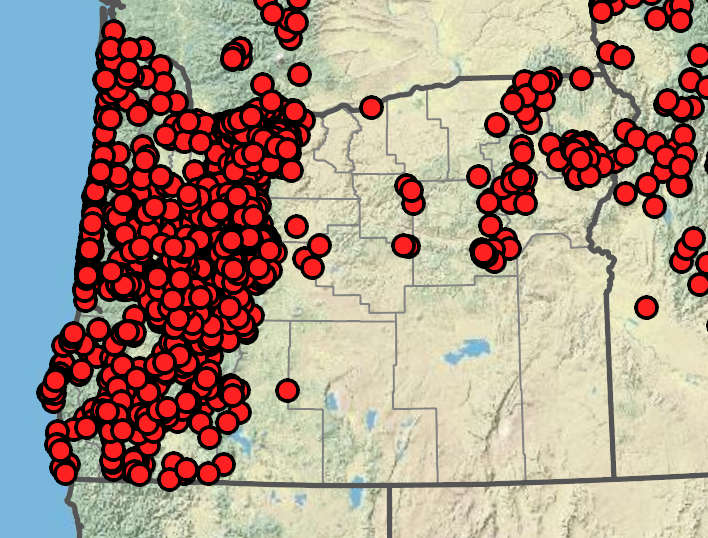
Oregon is home to three varieties of Pearly Everlasting whose native ranges extend the Cascade range, Western Oregon and parts of northeastern Oregon. Maps and legend acquired from the Oregon Flora Project, with Imagery Sourced from Google. Copyright 2021© TerraMetrics
Pearly Everlasting as a pollinator plant
Pearly Everlasting is an herbaceous perennial commonly seen in open meadows, burned areas, rocky flats and along roadsides in dry, sun-exposed soils. Native throughout the United States, except for the Southwest, Pearly everlasting is an excellent nectar resource for pollinators, and is especially attractive to many butterfly and moth species. It makes an important larval host plant for American Lady and Painted Lady Butterflies whose seasonal feeding can leave Pearly Everlasting foliage slightly tattered, but nothing that the plant can’t recover from.
Pearly Everlasting hosts a moderate abundance and a relatively low diversity of insect visitors, but is a key host plant for its associated pollinators.
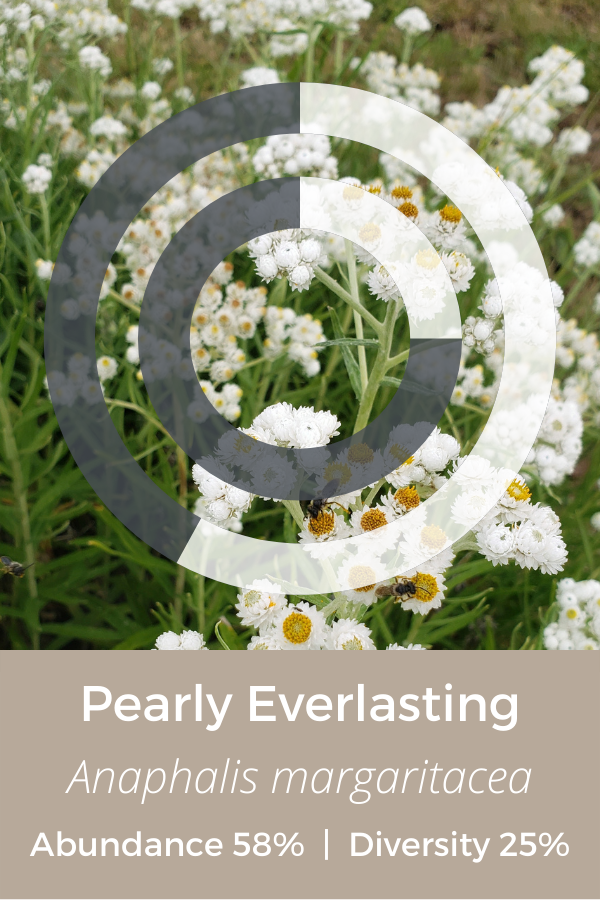
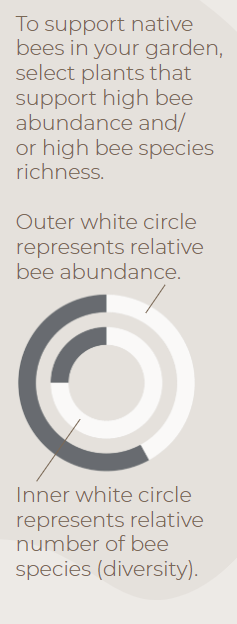
Infographics developed by LeAnn Locher, Aaron Anderson, and Gail Langellotto.
Abundance and Diversity Calculations. Bee abundance was calculated using estimated marginal means of bee visitation to each of our study plants from 5-minute observations conducted from Aaron’s 2017-2019 field seasons. Estimated marginal means (EM Means) were assigned to categorical values and averaged across years to yield the following categories: 0% = Very Low =EM mean below 0.49; 25% = Low = EM mean of 0.50 to 0.99; 50% = Moderate = EM mean of 1 to 1.49; 75% = High = EM mean of 1.50 to 1.99; and 100% = Very high = EM mean above 2.0.
Bee diversity was based on the total sum of species collected on each of our study plants from 2017 to 2019. A Chao 2 Estimator was used to estimate total expected species richness for each plant; Chao 2 estimates were then used to create categorical values, as follows: 0% = Very Low = 9.99 or lower; 25% = Low = 10 to 14.99; 50% = Moderate = 15 to 19.99; 75% = High = 20 to 24.99; 100% = Very high = 25 or higher.
Did you know?
As a plant that thrives in high light and very dry conditions, Pearly Everlasting is one of the first plants to colonize recently burned forests. When rain comes after a fire-season, Pearly Everlasting sends out rhizomes that allow the plant to spread rapidly across nutrient-rich areas. Similarly in a garden setting, Pearly Everlasting has low moisture and nutrient needs but when heavily watered and fertilized, it can quickly take over.
Established Pearly Everlasting should not be irrigated more than twice per month in the summer months. The white, petal-like bracts of Pearly Everlasting flowers retain a fresh appearance after being dried, so gardeners that allow aboveground growth to dry out in the summer months will be rewarded with dried flowers perfect for floral arrangements.
Photos from the field
Tune in next week for the next edition of our Pollinator Plant PR Campaign.