This is the second is a series of articles that I am writing for the Hardy Plant Society of Oregon Quarterly Magazine. I extend my thanks to the HPSO editorial team for improvements to my narrative.
***************
Humans benefit from the natural world in many ways. These benefits include the products (such as food, fiber, or timber) that we can harvest from nature, or the processes (such as pollination, biological control, or nutrient cycling) that make earth such a nice place to live. Whether people recognize the importance of these so-called “ecosystem services” to our health and well-being varies considerably as a function of education, past experiences with nature, and other socio-economic factors. In fact, some people find abundant ecosystem disservices in nature. For example, some people view natural areas as dangerous places that should be avoided.
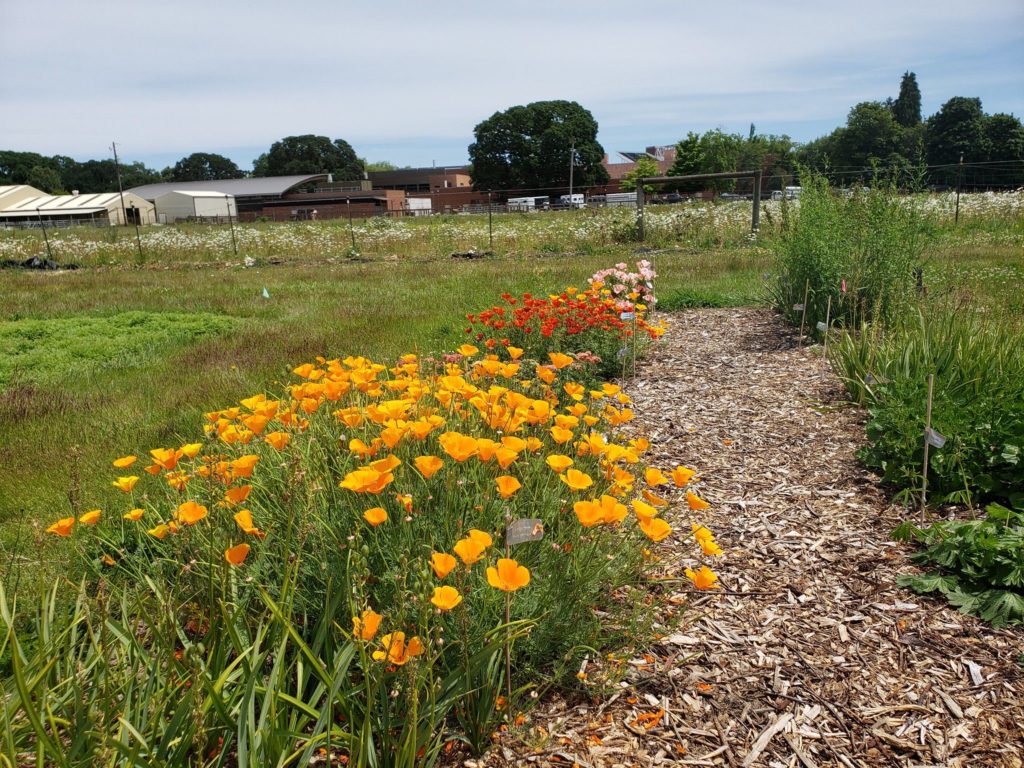
In an increasingly urbanized world, where many people lack meaningful interaction with the natural world, how can we help ensure that the importance of nature is recognized and valued? As Robert Michael Pyle wrote in his book The Thunder Tree: Lessons from an Urban Wildland (1998: Oregon State University Press), “What is the extinction of a condor to a child who has never seen a wren?”
One approach to helping society value the natural world is to put a dollar value on it, and that’s just what Robert Costanza and 12 colleagues did over the course of a five-day workshop hosted by the National Center for Ecological Analysis and Synthesis in 1996. A few months later, they published the second global accounting of the monetary value of the ecosystem goods and services of our planet1.
The authors found over 100 studies that valued one or more ecosystem services. They standardized the dollar value of each ecosystem service as the 1995 dollar value per hectare. They noted the location of each study and categorized the biome where the study occurred. They also generated novel estimates of the dollar value of various ecosystem services in various biomes by constructing what were essentially supply and demand curves. With these curves, they mathematically asked questions such as “How much more valuable would pollinators be, if they were endangered?” In this way, they were able to mathematically manipulate supply and demand curves and estimate what is known as the “marginal value” of each ecosystem service. In short, they used a lot of math. On a global map, they measured the area taken up by each biome. They multiplied the dollar value of each ecosystem service per unit area by the area taken up by each biome and developed a global map of sum-total value of ecosystem services.
The authors estimated that the value of the earth’s ecosystem services averaged $33 trillion dollars per year (1995 dollar value), which was 1.8 times the global gross national product. Nutrient cycling represented the highest valued ecosystem service, at $17 trillion per year. Coastal systems were identified as the most valuable biome, at $12 trillion per year.
Urban and suburban areas were included in the study. What struck me about this paper, however, was that the dollar value of ecosystem services of urban areas was not listed. Instead, the authors noted that ecosystem services in urban areas (like desert, rocks, tundra) “do not occur or are known to be negligible.”
When I read this paper as a young Ph.D. student in 1997, I was incensed. My family grew food and raised chickens and rabbits in the backyard of our Baltimore rowhouse (ecosystem service = food). As a child, I captured water striders, turtles, and tadpoles from urban streams (ecosystem service = habitat). As an undergraduate, I loved exploring the urban forests of Patapsco Valley State Park for exercise and stress management (ecosystem service = recreation). Did urban areas really deserve a zero? This paper made me want to study the ecosystem services of cities, just to prove the authors wrong!
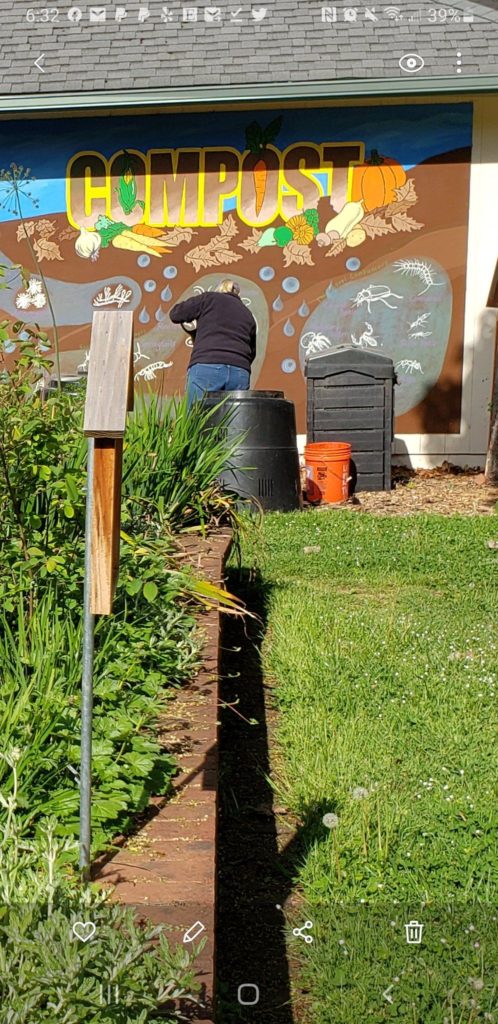
In 2002 I started to study the ecology of urban areas, as an assistant professor of biology at Fordham University in New York City. I collaborated with doctoral student Kevin Matteson to study the value of urban gardens as wildlife habitat and pollinator conservatories. We found that 18 small gardens dotting one of the most urbanized landscapes on earth were used by a diversity of insects, including 24 species of butterfly and 54 species of bee. At this same time, others were also documenting the ecosystem services of urban areas. For example, The New School’s Timon McPhearson estimated that raised bed gardens in New York City annually helped to retain and manage 12 million gallons of stormwater from flooding city streets. Karin Burghardt, as a University of Delaware undergraduate studying with Doug Tallamy, showed how plant choices can increase bird abundance and diversity in suburban gardens in Pennsylvania.
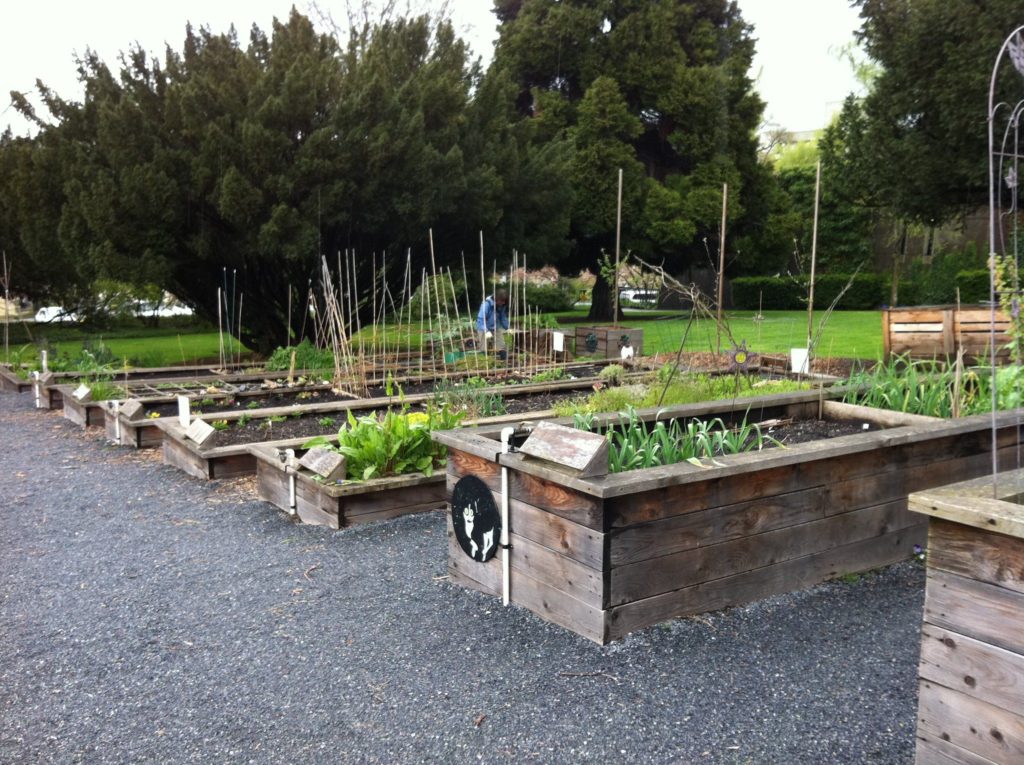
In fact, the early 2000s were a heyday for urban ecology research, due in large part to National Science Foundation funding of urban long-term ecological research efforts in Phoenix, Arizona, and Baltimore, Maryland. Whereas less than one-half of one percent of all papers published in nine leading ecological journals between 1995 and 2000 focused on urban systems or urban species (Collins et al. 2000), by 2016 over 1,000 articles, books, and book chapters have been published; and over 130 students have been trained in urban ecology by the Phoenix and Baltimore programs, alone (McPhearson et al. 2016). Despite these advances, the field of urban ecology is still relatively young, and much remains to be discovered.
In 2014, Costanza and colleagues published a new paper, with an updated estimate for the value of our globe’s ecosystem services.2 They estimated that natural systems annually provided $125 trillion (2011 US$ value) in ecosystem services to humanity. At least part of this increase is due to improved documentation of the portfolio of ecosystem services provided by different biomes (see table). And this time, ecosystem services provided by urban areas were valued at $2.3 trillion dollars, or $2.9 trillion in inflation-adjusted dollars for 2020.
”Hmph,” I thought. “At least it’s a start.”
1Robert Costanza et al. (1997): “The value of the world’s ecosystem services and natural capital.” Nature 387: 253-260.
2Costanza (2014): “Changes in the global value of ecosystem services.” Global Environmental Change 26: 152-158.
Table 1. The Value And Ecosystem Services Provided By Various Biomes On Earth. All dollar values have been inflation adjusted to 2020 dollar values, and are reported as TRILLIONS of dollars. Red Text: Identified as a service in Costanza et al., 1997, but not 2014; Green Text: Identified as a service in Costanza et al., 2014, but not 1997; Black Text: identified as a service across both papers.
Biome | Value from 1997 Paper | Value from 2014 Paper | Ecosystem Services |
Open Ocean | $14.8 | $27.9 | Gas regulation, Cultural, Climate regulation, Genetic resources, Recreation, Nutrient cycling, Biocontrol, Food |
Coastal | $22.1 | $35.3 | Climate regulation, Erosion control, Genetic resources, Disturbance regulation, Nutrient cycling, Biocontrol, Waste treatment, Habitat, Food, Raw materials, Recreation, Cultural |
Forest | $8.3 | $20.7 | Gas regulation, Pollination, Habitat, Climate regulation, Disturbance regulation, Water regulation, Water supply, Erosion control, Soil formation, Nutrient cycling, Waste treatment, Biocontrol, Food, Raw materials, Genetic resources, Recreation, Cultural |
Grassland | $1.5 | $23.5 | Climate regulation, Water supply, Habitat, Raw materials, Genetic resources, Cultural, Gas regulation, Water regulation, Erosion control, Soil formation, Waste treatment, Pollination, Biocontrol, Food, Recreation |
Wetlands | $8.5 | $33.65 | Gas regulation, Climate regulation, Erosion control, Nutrient cycling, Biocontrol, Genetic resources, Disturbance regulation, Water regulation, Waste treatment, Habitat, Food, Raw materials, Recreation, Cultural |
Lakes/Rivers | $2.9 | $3.1 | Water regulation, Water supply, Waste treatment, Food, Recreation |
Desert | $0 | $0 | |
Tundra | $0 | $0 | |
Ice/Rock | $0 | $0 | |
Cropland | $0.3 | $11.9 | Climate regulation, Water supply, Erosion control, Soil formation, Waste treatment, Raw materials, Genetic resources, Recreation, Pollination, Biocontrol, Food, |
Urban | $0 | $2.9 | Climate regulation, Water regulation, Recreation |