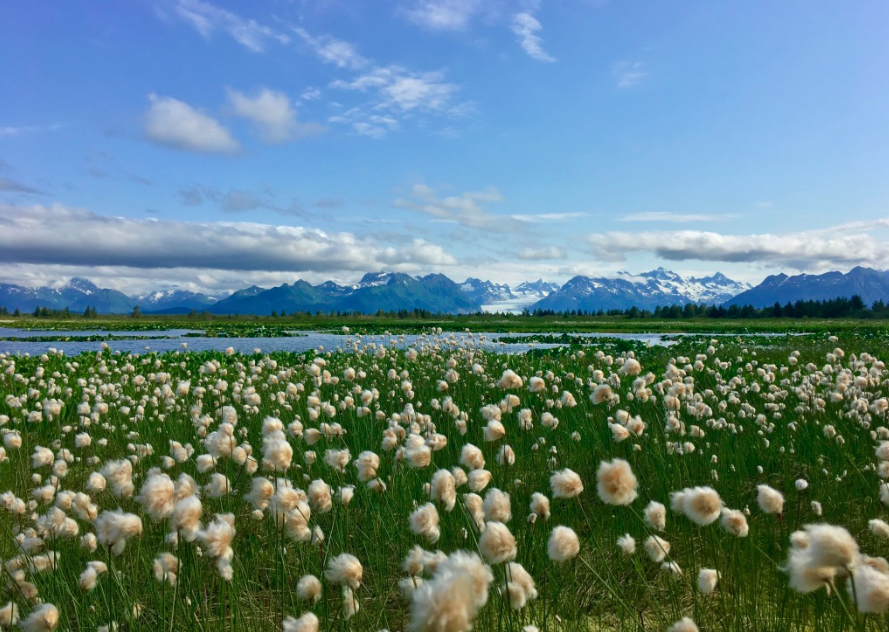
History of Glaciation Events
Earth has an ancient and romantic relationship with the greenhouse effect and glacier/ice sheet formation. When in the atmosphere, carbon dioxide (CO2) acts as a warm blanket around the Earth due to its ability to absorb energy from sun rays. The retention of this heat, in turn, warms the whole planet, known as the greenhouse effect, and these alterations in atmospheric CO2 affect global climate and glacier formation.
For example, 2.4-2.1 billion years ago geologic volcanic activity diminished, causing the amount of CO2 in the atmosphere to crash. This lost Earth its cosmic blanket, plunging the planet into a deep freeze, known as the Huronian Glaciation. Alternatively, biological events are also capable of altering Earth temperature, such as the evolution of photosynthesis and the eventual migration of multicellular plants on land. The sheer amount of photosynthesis occuring took vast volumes of CO2 out of the atmosphere, leading to the Cryogenian Glaciation 360-260 million years ago. The glaciers we know on Earth today formed recently during an ice age and numerous glaciation events beginning 2.58 million years ago during the Quaternary period, due to many factors from planetary orbit, to weathering of the Himalayas. Conversely, addition of CO2 to the atmosphere causes the Earth to warm, leading to interludes in glacier dominance, such as the Eocene Epoch. These warmer intermissions are known as “interglacial periods”, and are capable of occurring very rapidly due to “positive feedback loops”, or a self-perpetuating cycle; melting ice causes more ice to melt faster.
Alternating severe cooling and warming events are well documented in Earth’s history and directly correlate to the amount of CO2 in our atmosphere and relative sea level. Our present, the Holocene Epoch, is an unprecedented interglacial period due to “anthropogenic”, or human-derived, input from continuous burning fossil fuels beginning around the Industrial Revolution in the 19th and 20th centuries. Historic interglacial periods typically last 10,000 to 30,000 years, yet the Holocene interglacial period is projected to persist for 50,000+ years, assuming all CO2 levels are reduced to well BELOW pre industrial levels… a fairly unreasonable assumption. Historic biological and geochemical increases of CO2 into Earth’s atmosphere average 185 ppm, while amounts after the Industrial Revolution, a mere ~250 years ago, exceeded 390 ppm in the year 2010, and hover around 410ppm today.
Why Care about Glaciers?
Glaciers are freshwater reservoirs, presently containing approximately 2.1% of Earth’s water. That may not seem like a lot, but 97.2% of all water on Earth is salty. The remaining 0.7% freshwater is found in the atmosphere and all lakes, rivers, plants, and animals on Earth. By holding over thirty percent of all freshwater, glaciers act as safeguards of this precious resource. Seasonal melt events replenish downstream ecosystems, such as rivers, wetlands, and lakes, making water available for plants and animals. Glaciers also reserve minerals, nutrients, and organic compounds. The colloquial expression “glacial pace” comes about from the astonishingly slow movement of glaciers as frozen rivers grinding along mountain tops. This grinding action incorporates minerals such as iron from the mountain rocks, storing these resources in the ice. Seasonal/interglacial melt events release these minerals and nutrients into the ecosystem in a visual spectacle known as “glacial flour” (above), which is then consumed by microbes, plants, and animals downstream.
Additionally, glaciers aid in the regulation of global weather. They are essentially massive ice cubes that dominate portions of Earth that are capable of sustaining strong temperature gradients, compared to the warmer Equator or regional lowlands. Wind and ocean currents are formed in response to masses of air or water rushing from these different areas of extreme temperature, known as “convective currents”. Loss of the more massive, frigid, reservoirs contribute to the weakened movement of air and water, leading to unpredictable weather patterns and a cacophony of known and unknown changes, as ocean currents and air masses are stymied.
Modern Glacier Loss
Our modern climate as a result of increased atmospheric CO2 is causing glaciers all over the world to melt at historically exceptionally high rates. In 2017, 43% of all world-wide river basins containing glaciers experienced “peak melt”, or the time at which glacier freshwater input peaks and permanently declines to extinction. Estimating exact volumes of glacier loss around the globe is challenging, as glaciers vary greatly in size and geological location. Despite these challenges, researchers have been able to estimate that a decrease in global glacier volume will exceed 74 ± 11% by the end of the century. The remaining persistent glacial volume is largely located in the Himalayas around the Ganges, Brahmaputra, and Indus River watersheds, and in Southcentral Alaska around the Copper River watershed. Their persistence is attributed to a relatively large size in ice mass, lending them area to spare in these mass loss events.
Copper River Delta, Alaska, Research
In an effort to understand how the rapid melt of glaciers affects the ecosystem, Colwell Geomicrobiology Lab has been conducting ongoing field research in the Copper River Delta, AK (CRD) since 2017. We are focusing principally on how the nutrients and minerals found in the glacier meltwater change the microbes found in the downstream wetland sediments. The term subsurface refers to “below the Earth’s surface”, which is a whole unique ecosystem of microbes and molecules compared to surface ecosystems, since the subsurface usually has little or no oxygen. This lack of oxygen allows for the microbes to access and consume glacier minerals in unique ways, which may affect how carbon is processed in the area.
Of the minerals being deposited into the CRD, iron is the most visually prominent. Iron has many forms, the most well known being rust. Rust occurs when iron comes into contact with oxygen, giving it a vibrant orange-red color, commonly seen on old cars and gardening tools. The same process occurs in wetlands full of iron, but instead of cars and tools, the rocks and streambeds look rusty (above)! Once in the wetland, the rust from the glaciers may work its way into the mud of ponds and streams–into the subsurface–where there is no oxygen and millions of microbes.
We are hypothesizing that the microbes in the subsurface are able to use the rust to consume methane. Methane (CH4) is a common form of carbon found in the air, land, and water all around Earth in various amounts. The largest natural source of methane is well known to be wetlands, which contribute 217 Tg each year: the same weight as 700 blue whales! This is an incredible amount, but other natural sources, such as reactions in the troposphere, are able to consume 528 Tg each year: the same as 1,700 blue whales. However, as Earth’s climate is changing, more methane is being released into the atmosphere, where it acts as a greenhouse gas 3x stronger than CO2–more of a down comforter than a blanket. Human-sourced outputs of methane have increased significantly since the Industrial Revolution, the most common sources being ruminants (cow feedlots), landfills, fires, rice agriculture, and fossil fuels, totalling 1,100 blue whales (334 Tg per year). This increase has not been matched in consumption, leading to a buildup of about 334 Tg of methane in the atmosphere every year.
By understanding how the microbes use rust to process methane, we will be able to visualize methane production in wetlands in response to glacier loss: a direct result of climate change. To tackle this large, changing system, our lab uses DNA sequencing to “see” the microbes in the mud. We also use autonomous sampling instruments to continuously sample the methane in the ponds over a year. In the future, we will be able to merge our understanding of the microbes, methane, and rust in the subsurface of the CRD, leading to our ability to predict biological responses to geochemical changes in a math model. Use of a math model in ecosystem science is similar to being able to anticipate the ingredients and flavor outcome in a popular soup. For example, if I say “chicken noodle soup”, you are able to predict that particular soup has chicken, noodles, and even a few veggies like carrots and celery. Your ability to know the contents and taste of this soup comes from your past, fairly intimate, experience with eating the meal (possibly with some grilled cheese), and how it may have tasted differently given the ingredients you had on hand. The same goes for understanding the relationship between microbes, methane, and rust, the math model will make the prediction of the “flavor” combination based on past experiences (our previous research).
Rust to the Rescue by Jessica Buser-Young