How CEOAS scientists use DNA to study the tiniest aquatic organisms
By Nancy Steinberg
Fall/Winter 2022
Scene: the future. Vincent Freeman (played by Ethan Hawke) desperately wants to go to space but has been relegated to menial labor by a society that profiles and pigeonholes individuals based on their genetics. In order to assume another man’s genetic identity and realize his dream, Freeman uses a tiny vacuum device to remove all traces of his own DNA from his computer keyboard. Drama ensues.
Sound familiar? This is the classic sci-fi movie “Gattaca,” which envisions a future of DNA surveillance and profiling. For some scientists studying microscopic aquatic life, that future is here. These genetic traces are increasingly being used by CEOAS researchers to gain a wealth of information about unseen organisms, from bacteria to plankton. The resulting analyses help scientists figure out the composition, function and ecology of microbial and planktonic communities, leading to wider inferences about how aquatic ecosystems work.
Ghost DNA and what it reveals
Like Vincent Freeman, organisms of all kinds leave traces of DNA behind wherever they’ve been, originating from sloughed-off skin cells, fecal matter, mucus and other sources. Scientists can collect water samples and analyze these DNA ghosts to determine what species are or have been in an area of ocean. CEOAS oceanographer Maria Kavanaugh is chasing this so-called eDNA, or environmental DNA, one new component of her work on defining seascapes.
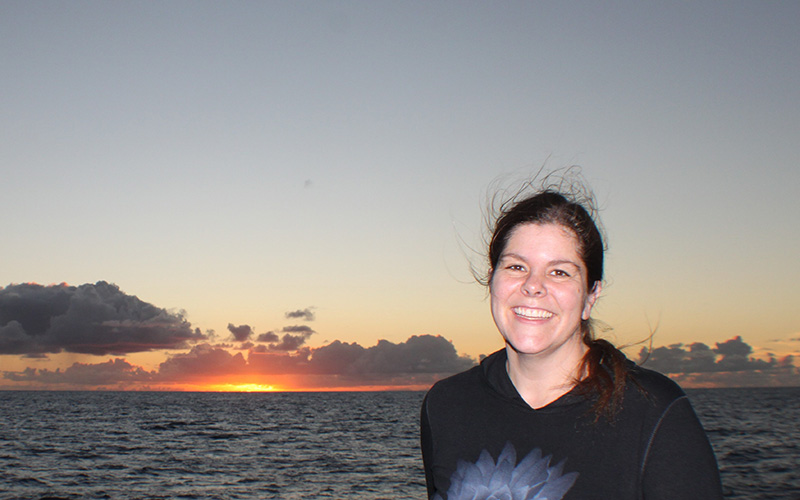
Maria Kavanaugh
Kavanaugh’s work on seascape ecology, inspired by the idea of landscape ecology, involves mapping regions in the ocean that have unique physical and biological characteristics and following how they change over time. Ultimately, an understanding of the gears that grind in a given habitat or seascape could improve our ability to predict and therefore manage marine resources.
With research partners at NOAA’s Northwest Fisheries Science Center, Kavanaugh is undertaking studies in the northern California Current ecosystem off the Oregon coast to contribute to seascape maps in the region, part of a global effort led by the Marine Biodiversity Observation Network. While eDNA can reveal the presence of any kind of organism, from microbes to whales, Kavanaugh would like to use it to focus on the planktonic animals and plants at the base of the food web.
There are two benefits, Kavanaugh says, to collecting and analyzing eDNA over other methods. “Bigger organisms, like some fish or krill, can be really patchy, so when we put out our nets, we may or may not capture what’s there, or what has been there. For the smaller stuff that I study, the genomic information [gathered from eDNA] can help us get to a higher taxonomic resolution than traditional oceanographic methods,” she says. In other words, eDNA offers the promise of creating a more complete picture of “who” is in a habitat by increasing both the likelihood of finding evidence of their presence and the ability to identify them to species level.
Kavanaugh and her team use DNA probes to determine what organisms contribute to the DNA soup in their water samples. Probes are short pieces of single-stranded DNA or RNA with a known sequence that search out their complementary piece in the sample and bind to it. Then the sample sequences are compared to massive databases of known organisms so individual species can be identified. Each taxonomic group requires its own set of analyses. If it sounds labor-intensive, Kavanaugh comments that it is not nearly as difficult as putting every water sample under a microscope to determine which species of plankton are present.
Coupled with other types of data collection, the species lists revealed by eDNA analysis will be able to help Kavanaugh map seascapes by showing where there are discontinuities in assemblages of species, meaning that sampling has crossed a “border” between one habitat and another. Species lists in a given area can be correlated with physical and chemical characteristics of the habitat to start to understand how ocean conditions may be impacting the base of the food web.
Who’s there?
Some CEOAS researchers are most interested in which species are present at the time that a water sample is collected, determined by extracting DNA from living organisms in that sample. When the research involves looking at the entire set of genomes present in a sample that contains many organisms, this technique is referred to as metagenomics.
Andrew Thurber uses this approach to characterize microbial communities in a variety of habitats including under Antarctic ice, on tropical coral reefs and at methane seeps off the Oregon and Washington coasts. He explains that advances in genetic techniques and bioinformatics — the critical science of analyzing massive, complex data sets like genetic codes — allow him to conduct research that was simply not possible a few decades ago. In those Dark Ages, like the early 1980s, species lists from an environmental sample were developed by growing species from the sample in a lab, but only a small fraction of microbes can be grown that way.
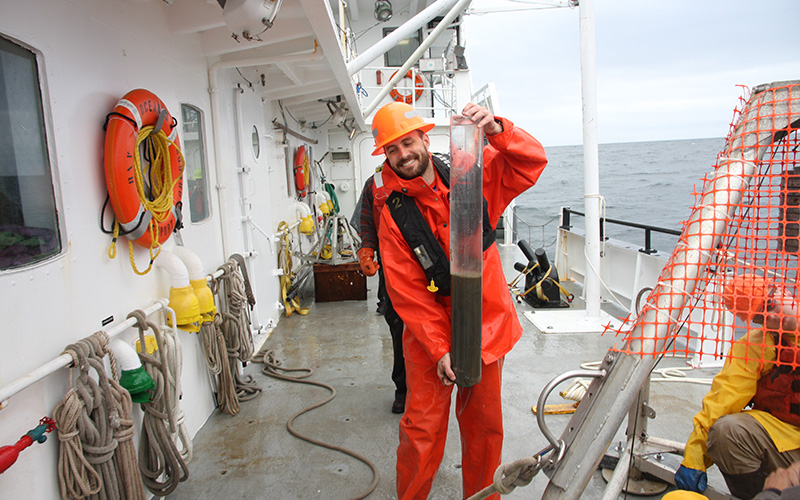
Andrew Thurber
Each sample Thurber and his lab submits to a bioinformatics lab comes back with a list of about 25 million DNA sequences. “What the brilliant people that are involved with bioinformatics have been able to do is look for patterns among millions or billions of short lengths of DNA. These clusters of DNA fragments allow us to construct the genomes of organisms that we cannot bring into culture and grow,” he says. “So, we can work in non-controlled systems in the field and get insight into the ecosystem. We can actually look at what the microbes are doing, and we can look at who the microbes are, including ones we might have been missing before.”
One place Thurber has applied these techniques is the Moorea Reef Long Term Ecological Research site in French Polynesia. On the island of Moorea, Thurber and collaborators have been examining microbial communities associated with corals in areas that are more and less impacted by human presence.
“There is a huge amount of spatial variability on this island. We wanted to know how variable the microbial community is in space, over time, and as a result of human impacts,” he explains. His team sampled corals over two and a half years, examining the microbial communities they found. “We found that every coral species had a different pattern of variation in their microbial community. Some were pretty constant and others seemed to change with how close they were to humans. We are currently teasing apart how this uniform vs. variable distribution allowed some of the species to survive a massive coral bleaching event, when certain areas experienced massive coral death and others much less. We are trying to use microbes and their DNA to understand what the reefs of the future will look like,” he says.
What are they doing?
Metagenomics allows scientists to take their queries one step further, asking not only who is present in a community, but what they’re doing there, or at least what they’re capable of doing. This kind of analysis cares less about the precise list of species in a sample and more about whether there are genes present that code for certain activities, like carbon or nitrogen fixation.
CEOAS microbial ecologist Byron Crump has used metagenomics to characterize the microbial community in Arctic coastal lagoons. He explains that these lagoons are changing quickly with the onset of climate change: “Significant warming in this area means the sea ice is melting sooner and maybe freezing later. The coastal permafrost is eroding into the lagoons, and the pace of that has increased.” He and his lab are finding a distinct seasonality to the microbial communities there, including bacteria, algae and protozoa. They will continue to track this community over time to determine how it is changing.
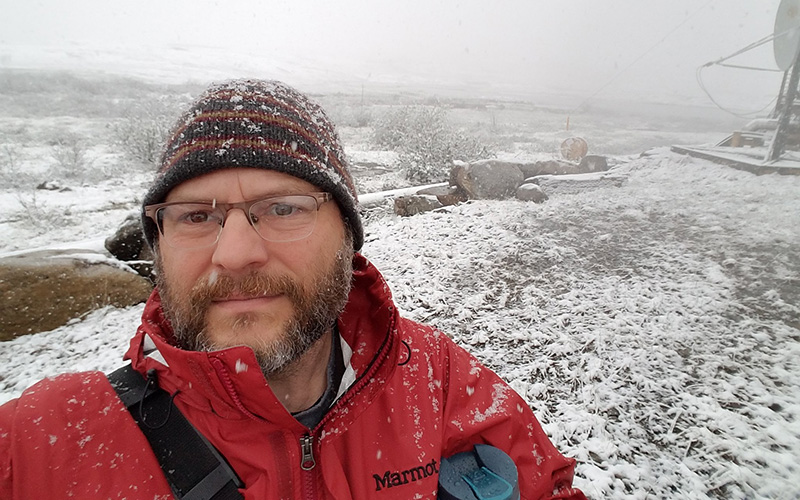
Byron Crump
Crump’s students, Christina Baker and Tasha Griffin, have used metagenomics to look for nitrifying genes — genes that help microorganisms use ammonia for fuel. Microbes that use nitrification to get energy usually thrive in the absence of sunlight; accordingly, Crump’s students found more nitrifying genes under the ice in the winter season (when sun cannot penetrate to the waters under the ice) than when the lagoons were ice-free.
“The total cell abundance during winter is one-tenth that of summer, but the abundance of nitrifying genes is significantly greater,” Crump says. “The organisms like phytoplankton that use light energy rather than ammonia can’t compete in this dark environment.” These results contribute to our understanding of a changing system, where ice cover is becoming scarcer as the system warms.
The real target: Everything, everywhere, all at once
The ultimate target of all of this work is to know more about microbial and phytoplankton community ecology, that is, understanding not just which species are present, but how they interact with their environment in space and time, especially as that environment changes. Crump and his students will pursue that goal by next looking at RNA, the molecule that reads the DNA code and turns it into working proteins, to know whether the genes present are “turned on.” Kavanaugh notes that seascapes are far from static, and climate change is bringing about unprecedented shifts in the ocean. Thurber is currently conducting RNA sampling at a new methane seep in the Antarctic to determine how methane-eating microbes have responded to emergence of the seep.
As technology advances, all of these analyses will likely become faster and cheaper, and these questions will become a little easier to address. More answers lie ahead, unless microbes learn to vacuum up their DNA behind them.