Amid COVID and wildfire related closures, finding a sense of order and normalcy in my research process has been challenging. Therefore, I consider myself lucky that I am able to continue the lab-based aspects of my PhD research. Lab work is time consuming and can be tedious, required days to months of repetitive tasks, but I am grateful for the order and organization that my lab workflow brings me. In this blog post, I’d like to share what this workflow looks like, the kind of information I gain from lab analyses, and some of my expected results from my last six months of lab work.
The workflow
After collecting, identifying, and measuring the length of hundreds of juvenile fishes using SMURFs (see my May blog post), it is time to take these fishes back to the lab. My lab work flow looks something like this: I use forceps and a scalpel to peel back the dorsal (top) of the fish’s cranium from the nose to the beginning of the vertebra tools to very thin forceps – the tips are as wide as 0.7mm mechanical pencil lead – and begin to move the brain away from the lateral sides of the cranium. I work under a dissecting microscope. My eyes look through the eye piece while my hands manipulate the specimen. I prod, search, and finally find what I’m so carefully looking for. I remove small white object from either side of the brain. They are slightly smaller than a grain of short grain rice. I try not to let my hands shake as I deposit the object safely in a microcentrifuge tube for storage, and take a breath of relief. 400 dissections later, I relocate to a new microscope station – a compound and dissecting scope with cameras that allow me to see and capture the microscope images on my computer. I mount each of these samples to a microscope slide, use 2000 grit sandpaper to sand both sides of the sample, and place it under 400X magnification. I smile – now the fun begins.
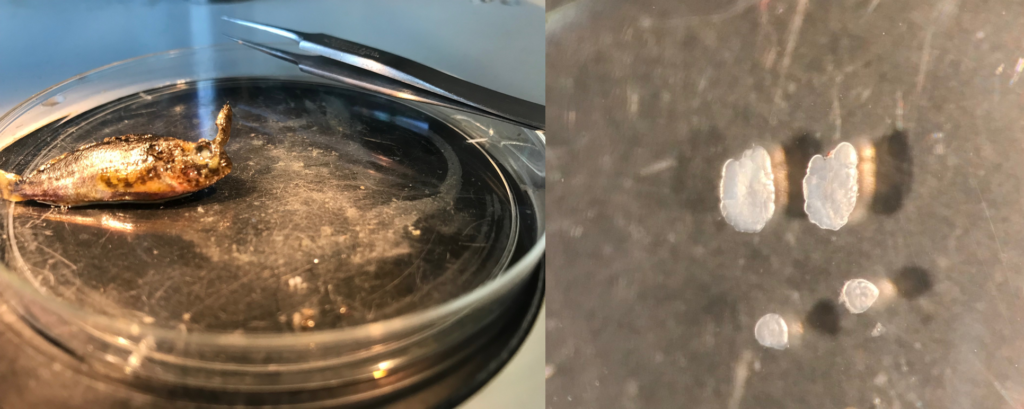
So why all this fuss? What are these mysterious small, nearly microscopic white objects?
Most fishes (except sharks, rays, and lamprey) have ear stones called otoliths, which are the small, white structures found in the cranium. Otoliths are made of calcium carbonate, the same compound found in the shells of many invertebrates. These structures that aid orientation, balance, and hearing, similar to human ear bones. Otoliths are an important tool in fish biology and fisheries science because they serve as an annual record book, kept over a fish’s entire life. Otoliths have alternating translucent and opaque zones that correspond to alternating periods of fast and slow growth, respectively. Otoliths have a familiar terrestrial analog: tree rings. I remember my fourth-grade teacher leading our class through a guided exploration of a tree “cookie”. We counted the rings to age the tree, observed alternating light and dark bands of different sizes, and connected these observations with seasonality (light availability, precipitation) and growth rates in nature (see my January blog post on seasonality in the ocean). Otoliths are a similarly powerful tool for fish biology and management, as they allow us to understand how fish populations grow and age over time and in relation to a changing climate, pollution, and even management regulations. They are also a powerful ecological tool – allowing scientists to peer into the lives of the fishes and understand what kinds of environmental conditions result in faster or slower growth.
For many years, fish biologists have used otoliths from adult fishes to measure population vital rates and translate this information into management decisions. In 1971, a groundbreaking discovery credited to Yale geologist Giorgio Panella forever changed the fields of fish biology, fish ecology, and fisheries science: it was possible to read the information stored in larval and juvenile fish otoliths as well – only in these young fishes, the alternating light and dark rings represented a daily log book. Imagine the excitement of scientists when they discovered that they could now peer into the daily lives of young fishes! In marine fishes, upwards of 99% of fish eggs produced do not survive to adulthood. There is a mortality gauntlet through which all young fish must pass, and few survive, due to the challenges of finding food in a vast open ocean, of avoiding myriad predators, and of avoiding currents that would sweep them away from suitable habitat. Prior to the discovery of otolith daily increments, this mortality gauntlet was poorly understood, making it difficult to predict when and why adult fish populations undergo periods of boom and bust.
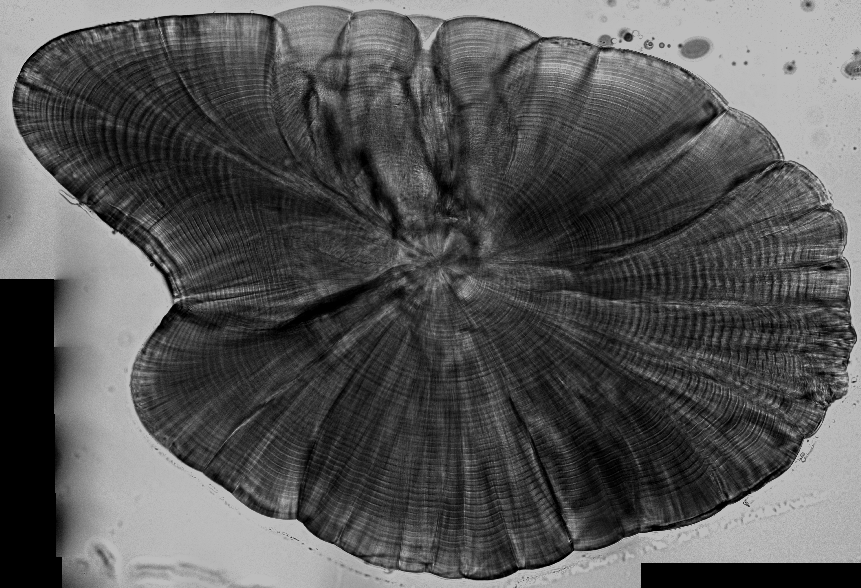
My research and expected results
Understanding this mortality gauntlet has been a central goal of my PhD research. Specifically, I study a socio-economically important nearshore groundfish found from Baja California, Mexico to Alaska, the cabezon (Scorpaenichthys marmoratus). Since COVID-19 made my 2020 field season impossible, I have instead been dissecting, polishing, and analyzing otolith data from juvenile cabezon with the goal of understanding what early life characteristics are important for growth and survival through the mortality gauntlet. Because the time series of samples for my project extend from 2013-2019, I am also able to investigate how these critical early life history characteristics change from year to year, and even from month to month.
Cabezon exhibit a somewhat unique recruitment strategy – that is, the timing and magnitude with which juveniles arrive to the nearshore to settle and grow into adulthood. Unlike many other nearshore groundfishes, cabezon recruit in multiple events, spanning the April – September months. This is a departure from the “single-pulse” strategy, where the juveniles (e.g. rockfishes) arrive over a short time window (e.g. two weeks in July). By the time the cabezon are collected in the SMURFs, they could be anywhere between 2 and 4 months old. That they arrive over a 6-month window means that they have experienced a vast range of ocean conditions (e.g. winter storms, changing currents, upwelling, downwelling, water temperature). I expect to find a “portfolio” of early life characteristics that enable the young fish to survive in different ocean conditions. For example, individuals that arrive to the SMURFs in May were likely hatched in January, experienced “winter” conditions, and could have a “winter” growing strategy (e.g. slow growth due to poor feeding conditions). In contrast, individuals that arrive to the SMURFs in July were likely hatched in April, experienced “spring” conditions, and could have a “spring” growing strategy (e.g. fast growth due to enhanced feeding conditions). Altogether, I am interested in understanding how this portfolio effect of early life strategies may enhance the resilience of the cabezon population to disturbances such as the 2014-2016 marine heat wave and other climate and fishing related changes.
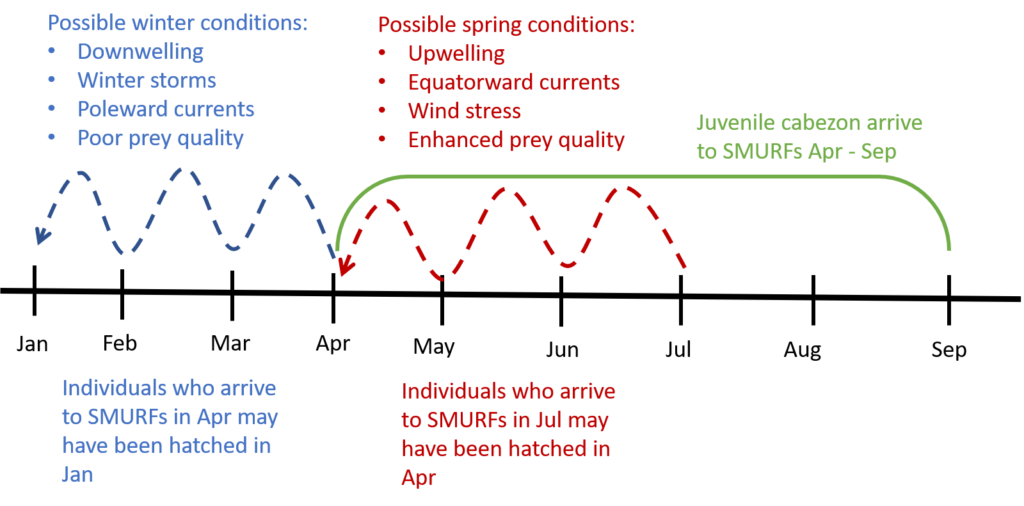